Environmental impact of pesticides

The environmental effects of pesticides describe the broad series of consequences of using pesticides. The unintended consequences of pesticides is one of the main drivers of the negative impact of modern industrial agriculture on the environment. Pesticides, because they are toxic chemicals meant to kill pest species, can affect non-target species, such as plants, animals and humans. Over 98% of sprayed insecticides and 95% of herbicides reach a destination other than their target species, because they are sprayed or spread across entire agricultural fields.[1] Other agrochemicals, such as fertilizers, can also have negative effects on the environment.
The negative effects of pesticides are not just in the area of application. Runoff and pesticide drift can carry pesticides into distant aquatic environments or other fields, grazing areas, human settlements and undeveloped areas. Other problems emerge from poor production, transport, storage and disposal practices.[2] Over time, repeat application of pesticides increases pest resistance, while its effects on other species can facilitate the pest's resurgence.[3] Alternatives to heavy use of pesticides, such as integrated pest management, and sustainable agriculture techniques such as polyculture mitigate these consequences, without the harmful toxic chemical application.
Environmental modelling indicates that globally over 60% of global agricultural land (~24.5 million km²) is "at risk of pesticide pollution by more than one active ingredient", and that over 30% is at "high risk" of which a third are in high-biodiversity regions.[4][5] Each pesticide or pesticide class comes with a specific set of environmental concerns. Such undesirable effects have led many pesticides to be banned, while regulations have limited and/or reduced the use of others. The global spread of pesticide use, including the use of older/obsolete pesticides that have been banned in some jurisdictions, has increased overall.[6][7]
Natural Pesticides
Chemical Pesticides have been used for years, this is due to the immediate benefits that agribusiness gets. From their cost effectiveness to their assistance in decreasing disease spread, and increasing crop production, these pesticides appear to be a great resource. However, studies have shown that chemical pesticides can cause serious issues for those that consume them and the environment around them.[8] They can destroy ecosystems and introduce all sorts of toxins to the people and animals that consume them. Even the non-active ingredients that are often assumed to be non-toxic can be the very toxic ingredient that causes harm. Perhaps, the most commonly seen issue with pesticides is their effect on pollinators.[8] While designed to kill pests, these chemicals also tend to kill or damage the very creatures that keep agriculture going. Sure, some chemical pesticides are mild enough to not harm the good insects, but all it takes is human error to make what’s harmless, harmful. Some effects are unknown due to the complicated and difficult to predict effect of combining pesticides. In some instances, natural pesticides offer a better alternative for farmers.[9] Natural pesticides are pesticides that are found in nature and made from minerals, plants, and microorganisms. While they do not last as long as synthetic pesticides and are less toxic, they are more environmentally safe. (This does not mean they are safe for humans, as they are still meant to kill insects.) Specific types of natural pesticides include botanical pesticides- natural occurring chemicals extracted directly from plants or minerals; biochemical pesticides- uses substances like plant hormones that could interfere with pests mating or other behaviors; and microbial pesticides- pesticide with microorganisms like fungi, viruses, or bacteria as the active ingredient. Other things such as rosemary, marigolds, chrysanthemums, lavender, and basil work as a natural deterrent against specific pests.[10]
History
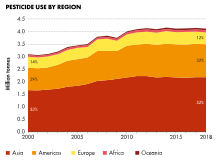
The first synthetic herbicides were discovered in the 1930's and 1940's. This was the era when synthetic antibiotics, plastics, and many other materials became available. Synthetic pesticides became popular rapidly after World War II. Crop yields increased significantly through the discovery of 2,4-D.[11][12][13][14] Many insect infestations were addressed by DDT, greatly lowering rates of typhus and malaria worldwide. In 1962, an estimated 85,000,000 kilograms of DDT was produced in the US alone.[15]
Public concern over the undesirable environmental effects of chemicals arose in the early 1960s with the publication of Rachel Carson′s book, Silent Spring. Shortly thereafter, DDT, originally used to combat malaria, and its metabolites were shown to cause population-level effects in raptorial birds. Initial studies in industrialized countries focused on acute mortality effects mostly involving birds or fish.[16]
Modern pesticide usage
Today, over 3.5 billion kilograms of synthetic pesticides are used for the world's agriculture in an over $45 billion industry.[17] Current lead agrichemical producers include Syngenta (ChemChina), Bayer Crop Science, BASF, Dow AgroSciences, FMC, ADAMA, Nufarm, Corteva, Sumitomo Chemical, UPL, and Huapont Life Sciences. Bayer CropScience and its acquisition of Monsanto led it to record profits in 2019 of over $10 billion in sales, which herbicide shares growing by 22%, followed closely by Syngenta.[18]
In 2016, the United States consumed 322 million pounds (146,056,743 kg) of pesticides banned in the EU, 26 million pounds (11,793,402 kg) of pesticides banned in Brazil and 40 million pounds (18,143,695 kg) of pesticides banned in China, with most of banned pesticides banned staying constant or increasing in the United States over the past 25 years according to studies.[19]
In the United States, conventional pesticide use peaked in 1979, and by 2007, had been reduced by 25 percent from the 1979 peak level,[20] while US agricultural output increased by 43 percent over the same period.[21]
Specific pesticide effects
Pesticide/class | Effect(s) |
---|---|
Organochlorine DDT/DDE | Endocrine disruptor[22] |
Thyroid disruption properties in rodents, birds, amphibians and fish[23] | |
Acute mortality attributed to inhibition of acetylcholinesterase activity[24] | |
DDT | Egg shell thinning in raptorial birds[23] |
Carcinogen[22] | |
Endocrine disruptor[22] | |
DDT/Diclofol, Dieldrin and Toxaphene | Juvenile population decline and adult mortality in wildlife reptiles[25] |
DDT/Toxaphene/Parathion | Susceptibility to fungal infection[26] |
Triazine | Earthworms became infected with monocystid gregarines[16] |
Chlordane | Interact with vertebrate immune systems[26] |
Carbamates, the phenoxy herbicide 2,4-D, and atrazine | Interact with vertebrate immune systems[26] |
Anticholinesterase | Bird poisoning[24] |
Animal infections, disease outbreaks and higher mortality.[27] | |
Organophosphate | Thyroid disruption properties in rodents, birds, amphibians and fish[23] |
Acute mortality attributed to inhibition of acetylcholine esterase activity[24] | |
Immunotoxicity, primarily caused by the inhibition of serine hydrolases or esterases[28] | |
Oxidative damage[28] | |
Modulation of signal transduction pathways[28] | |
Impaired metabolic functions such as thermoregulation, water and/or food intake and behavior, impaired development, reduced reproduction and hatching success in vertebrates.[29] | |
Carbamate | Thyroid disruption properties in rodents, birds, amphibians and fish[23] |
Impaired metabolic functions such as thermoregulation, water and/or food intake and behavior, impaired development, reduced reproduction and hatching success in vertebrates.[29] | |
Interact with vertebrate immune systems[26] | |
Acute mortality attributed to inhibition of acetylcholine esterase activity[24] | |
Phenoxy herbicide 2,4-D | Interact with vertebrate immune systems[26] |
Atrazine | Interact with vertebrate immune systems[26] |
Reduced northern leopard frog (Rana pipiens) populations because atrazine killed phytoplankton, thus allowing light to penetrate the water column and periphyton to assimilate nutrients released from the plankton. Periphyton growth provided more food to grazers, increasing snail populations, which provide intermediate hosts for trematode.[30] | |
Pyrethroid | Thyroid disruption properties in rodents, birds, amphibians and fish[23] |
Thiocarbamate | Thyroid disruption properties in rodents, birds, amphibians and fish[23] |
Triazine | Thyroid disruption properties in rodents, birds, amphibians and fish[23] |
Triazole | Thyroid disruption properties in rodents, birds, amphibians and fish[23] |
Impaired metabolic functions such as thermoregulation, water and/or food intake and behavior, impaired development, reduced reproduction and hatching success in vertebrates. | |
Neonicotinoic/Nicotinoid | respiratory, cardiovascular, neurological, and immunological toxicity in rats and humans[31] |
Disrupt biogenic amine signaling and cause subsequent olfactory dysfunction, as well as affecting foraging behavior, learning and memory. | |
Imidacloprid, Imidacloprid/pyrethroid λ-cyhalothrin | Impaired foraging, brood development, and colony success in terms of growth rate and new queen production.[32] |
Thiamethoxam | High honey bee worker mortality due to homing failure[33] (risks for colony collapse remain controversial)[34] |
Flupyradifurone | Lethal and sublethal adverse synergistic effects in bees.[35] Its toxicity depends on season and nutritional stress, and can reduce bee survival, food consumption, thermoregulation, flight success, and increase flight velocity.[36] It has the same mode of action of neonicotinoids.[37] |
Spinosyns | Affect various physiological and behavioral traits of beneficial arthropods, particularly hymenopterans[38] |
Bt corn/Cry | Reduced abundance of some insect taxa, predominantly susceptible Lepidopteran herbivores as well as their predators and parasitoids.[16] |
Herbicide | Reduced food availability and adverse secondary effects on soil invertebrates and butterflies[39] |
Decreased species abundance and diversity in small mammals.[39] | |
Benomyl | Altered the patch-level floral display and later a two-thirds reduction of the total number of bee visits and in a shift in the visitors from large-bodied bees to small-bodied bees and flies[40] |
Herbicide and planting cycles | Reduced survival and reproductive rates in seed-eating or carnivorous birds[41] |
Persistent organic pollutants
Persistent organic pollutants (POPs) are compounds that resist degradation and thus remain in the environment beyond their intended term. Some pesticides, including aldrin, chlordane, DDT, dieldrin, endrin, heptachlor, hexachlorobenzene, mirex, and toxaphene, are considered POPs. Some POPs have the ability to volatilize and travel great distances through the atmosphere to become deposited in remote regions. Such chemicals may have the ability to bioaccumulate and biomagnify and can biomagnify (i.e. become more concentrated) up to 70,000 times their original concentrations.[42] POPs can affect non-target organisms in the environment and increase risk to humans[43] by disruption in the endocrine, reproductive, and respiratory systems.[42]
The half-life of pesticides is of great interest. Some pesticides degrade rapidly in the environment, others, called persistent, linger, causing undesired effects.[44] and certain authors maintain that pesticide risk and impact assessment models rely on and are sensitive to information describing dissipation from plants.[45]
The half-life for pesticides is explained in two NPIC fact sheets. Known degradation pathways are through: photolysis, chemical dissociation, sorption, bioaccumulation and plant or animal metabolism.[46][47] A USDA fact sheet published in 1994 lists the soil adsorption coefficient and soil half-life for then-commonly used pesticides.[48][49]
Environmental effects
Air

Pesticides contribute to air pollution. Pesticide drift occurs when pesticides suspended in the air as particles are carried by wind to other areas, potentially contaminating them.[50] Pesticides that are applied to crops can volatilize and may be blown by winds into nearby areas, potentially posing a threat to wildlife.[51] Weather conditions at the time of application as well as temperature and relative humidity change the spread of the pesticide in the air. As wind velocity increases so does the spray drift and exposure. Low relative humidity and high temperature result in more spray evaporating. The amount of inhalable pesticides in the outdoor environment is therefore often dependent on the season.[3] Also, droplets of sprayed pesticides or particles from pesticides applied as dusts may travel on the wind to other areas,[52] or pesticides may adhere to particles that blow in the wind, such as dust particles.[53] Ground spraying produces less pesticide drift than aerial spraying does.[54] Farmers can employ a buffer zone around their crop, consisting of empty land or non-crop plants such as Evergreen trees to serve as windbreaks and absorb the pesticides, preventing drift into other areas.[55] Such windbreaks are legally required in the Netherlands.[55]
Pesticides that are sprayed on to fields and used to fumigate soil can give off chemicals called volatile organic compounds, which can react with other chemicals and form a pollutant called ground level ozone. Pesticide use accounts for about 6 percent of total ground level ozone levels.[56]
Water
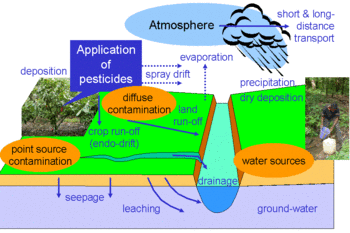
In the United States, pesticides were found to pollute every stream and over 90% of wells sampled in a study by the US Geological Survey.[57] Pesticide residues have also been found in rain and groundwater.[58] Studies by the UK government showed that pesticide concentrations exceeded those allowable for drinking water in some samples of river water and groundwater.[59]
Pesticide impacts on aquatic systems are often studied using a hydrology transport model to study movement and fate of chemicals in rivers and streams. As early as the 1970s quantitative analysis of pesticide runoff was conducted to predict amounts of pesticide that would reach surface waters.[60]
There are four major routes through which pesticides reach the water: it may drift outside of the intended area when it is sprayed, it may percolate, or leach through the soil, it may be carried to the water as runoff, or it may be spilled, for example accidentally or through neglect.[61] They may also be carried to water by eroding soil.[62] Factors that affect a pesticide's ability to contaminate water include its water solubility, the distance from an application site to a body of water, weather, soil type, presence of a growing crop, and the method used to apply the chemical.[63]
Water-focused regulations
In United States regulation, maximum limits of allowable conce[64] ntrations for individual pesticides in drinking water are set by the Environmental Protection Agency (EPA) for public water systems.[58][63] (There are no federal standards for private wells.) Ambient water quality standards for pesticide concentrations in water bodies are principally developed by state environmental agencies, with EPA oversight. These standards may be issued for individual water bodies, or may apply statewide.[65][66]
The United Kingdom sets Environmental Quality Standards (EQS), or maximum allowable concentrations of some pesticides in bodies of water above which toxicity may occur.[67]
The European Union regulates maximum concentrations of pesticides in water.[67]
Soil
The extensive use of pesticides in agricultural production can degrade and damage the community of microorganisms living in the soil, particularly when these chemicals are overused or misused as chemical compounds build up in the soil.[68] The full impact of pesticides on soil microorganisms is still not entirely understood; many studies have found deleterious effects of pesticides on soil microorganisms and biochemical processes, while others have found that the residue of some pesticides can be degraded and assimilated by microorganisms.[69] The effect of pesticides on soil microorganisms is impacted by the persistence, concentration, and toxicity of the applied pesticide, in addition to various environmental factors.[70] This complex interaction of factors makes it difficult to draw definitive conclusions about the interaction of pesticides with the soil ecosystem. In general, long-term pesticide application can disturb the biochemical processes of nutrient cycling.[69]
Many of the chemicals used in pesticides are persistent soil contaminants, whose impact may endure for decades and adversely affect soil conservation.[71]
The use of pesticides decreases the general biodiversity in the soil. Not using the chemicals results in higher soil quality,[72] with the additional effect that more organic matter in the soil allows for higher water retention.[58] This helps increase yields for farms in drought years, when organic farms have had yields 20-40% higher than their conventional counterparts.[73] A smaller content of organic matter in the soil increases the amount of pesticide that will leave the area of application, because organic matter binds to and helps break down pesticides.[58]
Degradation and sorption are both factors which influence the persistence of pesticides in soil. Depending on the chemical nature of the pesticide, such processes control directly the transportation from soil to water, and in turn to air and our food. Breaking down organic substances, degradation, involves interactions among microorganisms in the soil. Sorption affects bioaccumulation of pesticides which are dependent on organic matter in the soil. Weak organic acids have been shown to be weakly sorbed by soil, because of pH and mostly acidic structure. Sorbed chemicals have been shown to be less accessible to microorganisms. Aging mechanisms are poorly understood but as residence times in soil increase, pesticide residues become more resistant to degradation and extraction as they lose biological activity.[74]
Impacts
Plants

Nitrogen fixation, which is required for the growth of vascular ("higher") plants, is hindered by pesticides in soil.[75] The insecticides DDT, methyl parathion, and especially pentachlorophenol have been shown to interfere with legume-rhizobium chemical signaling.[75] Reduction of this symbiotic chemical signaling results in reduced nitrogen fixation and thus reduced crop yields.[75] Root nodule formation in these plants saves the world economy $10 billion in synthetic nitrogen fertilizer every year.[76]
On the other side, pesticides have some direct harmful effect on plant including poor root hair development, shoot yellowing and reduced plant growth.[77]
Pollinators
Pesticides can kill bees and are strongly implicated in pollinator decline,[78] the loss of species that pollinate plants, including through the mechanism of Colony Collapse Disorder,[79][80][81][82][83][unreliable source?] in which worker bees from a beehive or western honey bee colony abruptly disappear. Application of pesticides to crops that are in bloom can kill honeybees,[50] which act as pollinators. The USDA and USFWS estimate that US farmers lose at least $200 million a year from reduced crop pollination because pesticides applied to fields eliminate about a fifth of honeybee colonies in the US and harm an additional 15%.[1]
Animals

Animal studies mostly focus on fish, insects, birds, amphibians and arachnids.[16] Many kinds of animals are harmed by pesticides, leading many countries to regulate pesticide usage through Biodiversity Action Plans. Animals including humans may be poisoned by pesticide residues that remain on food, for example when wild animals enter sprayed fields or nearby areas shortly after spraying.[54]
Pesticides can eliminate some animals' essential food sources, causing the animals to relocate, change their diet or starve. Residues can travel up the food chain; for example, birds can be harmed when they eat insects and worms that have consumed pesticides.[50] Earthworms digest organic matter and increase nutrient content in the top layer of soil. They protect human health by ingesting decomposing litter and serving as bioindicators of soil activity. Pesticides have had harmful effects on growth and reproduction on earthworms.[84] Some pesticides can bioaccumulate, or build up to toxic levels in the bodies of organisms that consume them over time, a phenomenon that impacts species high on the food chain especially hard.[50]
Birds

The US Fish and Wildlife Service estimates that 72 million birds are killed by pesticides in the United States each year.[86] Bald eagles are common examples of nontarget organisms that are impacted by pesticide use. Rachel Carson's book Silent Spring uncovered the effects of bioaccumulation of the pesticide DDT in 1962.
Farmland birds are declining more rapidly than birds of any other biome in North America, a decline that is correlated with intensification and expansion of pesticide usage.[87] In the farmland of the United Kingdom, populations of ten different bird species declined by 10 million breeding individuals between 1979 and 1999, allegedly from loss of plant and invertebrate species on which the birds feed. Throughout Europe, 116 species of birds were threatened as of 1999. Reductions in bird populations have been found to be associated with times and areas in which pesticides are used.[88] DDE-induced egg shell thinning has especially affected European and North American bird populations.[89] From 1990 to 2014 the number of common farmland birds has declined in the European Union as a whole and in France, Belgium and Sweden; in Germany, which relies more on organic farming and less on pesticides the decline has been slower; in Switzerland, which does not rely much on intensive agriculture, after a decline in the early 2000s the level has returned to the one of 1990.[85]
In another example, some types of fungicides used in peanut farming are only slightly toxic to birds and mammals, but may kill earthworms, which can in turn reduce populations of the birds and mammals that feed on them.[54]
Some pesticides come in granular form. Wildlife may eat the granules, mistaking them for grains of food. A few granules of a pesticide may be enough to kill a small bird.[54] Herbicides may endanger bird populations by reducing their habitat.[54] Furthermore, destruction of native habitat and conversion into other land-use types (e.g. agricultural, residential) contributes to the decline of these birds. Avicides poses a huge threat of direct poisoning of non-target birds. As poisoned birds can fly long distances before they die, death of non-target birds often remains unnoticed. Many countries have no registered pesticides of this group at all. In USA registered avicides belong to restricted use pesticides and can be used only by certified pest control operations.
Aquatic life


Fish and other aquatic biota may be harmed by pesticide-contaminated water.[90] Pesticide surface runoff into rivers and streams can be highly lethal to aquatic life, sometimes killing all the fish in a particular stream.[91]
Application of herbicides to bodies of water can cause fish kills when the dead plants decay and consume the water's oxygen, suffocating the fish. Herbicides such as copper sulfate that are applied to water to kill plants are toxic to fish and other water animals at concentrations similar to those used to kill the plants. Repeated exposure to sublethal doses of some pesticides can cause physiological and behavioral changes that reduce fish populations, such as abandonment of nests and broods, decreased immunity to disease and decreased predator avoidance.[90]
Application of herbicides to bodies of water can kill plants on which fish depend for their habitat.[90]
Pesticides can accumulate in bodies of water to levels that kill off zooplankton, the main source of food for young fish.[92] Pesticides can also kill off insects on which some fish feed, causing the fish to travel farther in search of food and exposing them to greater risk from predators.[90]
The faster a given pesticide breaks down in the environment, the less threat it poses to aquatic life. Insecticides are typically more toxic to aquatic life than herbicides and fungicides.[90]
Amphibians
In the past several decades, amphibian populations have declined across the world, for unexplained reasons which are thought to be varied but of which pesticides may be a part.[93]
Pesticide mixtures appear to have a cumulative toxic effect on frogs. Tadpoles from ponds containing multiple pesticides take longer to metamorphose and are smaller when they do, decreasing their ability to catch prey and avoid predators.[94] Exposing tadpoles to the organochloride endosulfan at levels likely to be found in habitats near fields sprayed with the chemical kills the tadpoles and causes behavioral and growth abnormalities.[95]
The herbicide atrazine can turn male frogs into hermaphrodites, decreasing their ability to reproduce.[94] Both reproductive and nonreproductive effects in aquatic reptiles and amphibians have been reported. Crocodiles, many turtle species and some lizards lack sex-distinct chromosomes until after fertilization during organogenesis, depending on temperature. Embryonic exposure in turtles to various PCBs causes a sex reversal. Across the United States and Canada disorders such as decreased hatching success, feminization, skin lesions, and other developmental abnormalities have been reported.[89]

Humans
Pesticides can enter the body through inhalation of aerosols, dust and vapor that contain pesticides; through oral exposure by consuming food/water; and through skin exposure by direct contact.[96] Pesticides secrete into soils and groundwater which can end up in drinking water, and pesticide spray can drift and pollute the air.
The effects of pesticides on human health depend on the toxicity of the chemical and the length and magnitude of exposure.[97] Farm workers and their families experience the greatest exposure to agricultural pesticides through direct contact. Every human contains pesticides in their fat cells.
Children are more susceptible and sensitive to pesticides,[96] because they are still developing and have a weaker immune system than adults. Children may be more exposed due to their closer proximity to the ground and tendency to put unfamiliar objects in their mouth. Hand to mouth contact depends on the child's age, much like lead exposure. Children under the age of six months are more apt to experience exposure from breast milk and inhalation of small particles. Pesticides tracked into the home from family members increase the risk of exposure. Toxic residue in food may contribute to a child's exposure.[98] Epidemiological studies have reported adverse effects of certain pesticides at current levels of exposure on children's cognitive development.[99] The chemicals can bioaccumulate in the body over time.
Exposure effects can range from mild skin irritation to birth defects, tumors, genetic changes, blood and nerve disorders, endocrine disruption, coma or death.[97] Developmental effects have been associated with pesticides. Recent increases in childhood cancers in throughout North America, such as leukemia, may be a result of somatic cell mutations.[100] Insecticides targeted to disrupt insects can have harmful effects on mammalian nervous systems. Both chronic and acute alterations have been observed in exposes. DDT and its breakdown product DDE disturb estrogenic activity and possibly lead to breast cancer. Fetal DDT exposure reduces male penis size in animals and can produce undescended testicles. Pesticide can affect fetuses in early stages of development, in utero and even if a parent was exposed before conception. Reproductive disruption has the potential to occur by chemical reactivity and through structural changes.[101]
Pest resistance
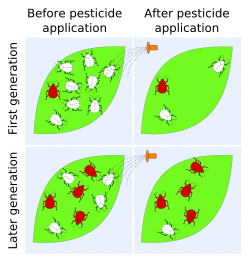
Pesticide resistance describes the decreased susceptibility of a pest population to a pesticide that was previously effective at controlling the pest. Pest species evolve pesticide resistance via natural selection: the most resistant specimens survive and pass on their acquired heritable changes traits to their offspring.[102] If a pest has resistance then that will reduce the pesticide's efficacy – efficacy and resistance are inversely related.[103]
Cases of resistance have been reported in all classes of pests (i.e. crop diseases, weeds, rodents, etc.), with 'crises' in insect control occurring early-on after the introduction of pesticide use in the 20th century. The Insecticide Resistance Action Committee (IRAC) definition of insecticide resistance is 'a heritable change in the sensitivity of a pest population that is reflected in the repeated failure of a product to achieve the expected level of control when used according to the label recommendation for that pest species'.[104]
Pesticide resistance is increasing. Farmers in the US lost 7% of their crops to pests in the 1940s; over the 1980s and 1990s, the loss was 13%, even though more pesticides were being used.[102] Over 500 species of pests have evolved a resistance to a pesticide.[105] Other sources estimate the number to be around 1,000 species since 1945.[106]
Although the evolution of pesticide resistance is usually discussed as a result of pesticide use, it is important to keep in mind that pest populations can also adapt to non-chemical methods of control. For example, the northern corn rootworm (Diabrotica barberi) became adapted to a corn-soybean crop rotation by spending the year when the field is planted with soybeans in a diapause.[107]
As of 2014, few new weed killers are near commercialization, and none with a novel, resistance-free mode of action.[108] Similarly, as of January 2019 discovery of new insecticides is more expensive and difficult than ever.[109]Pest rebound and secondary pest outbreaks
Non-target organisms can also be impacted by pesticides. In some cases, a pest insect that is controlled by a beneficial predator or parasite can flourish should an insecticide application kill both pest and beneficial populations. A study comparing biological pest control and pyrethroid insecticide for diamondback moths, a major cabbage family insect pest, showed that the pest population rebounded due to loss of insect predators, whereas the biocontrol did not show the same effect.[110] Likewise, pesticides sprayed to control mosquitoes may temporarily depress mosquito populations, they may result in a larger population in the long run by damaging natural controls.[50] This phenomenon, wherein the population of a pest species rebounds to equal or greater numbers than it had before pesticide use, is called pest resurgence and can be linked to elimination of its predators and other natural enemies.[111]
Loss of predator species can also lead to a related phenomenon called secondary pest outbreaks, an increase in problems from species that were not originally a problem due to loss of their predators or parasites.[111] An estimated third of the 300 most damaging insects in the US were originally secondary pests and only became a major problem after the use of pesticides.[1] In both pest resurgence and secondary outbreaks, their natural enemies were more susceptible to the pesticides than the pests themselves, in some cases causing the pest population to be higher than it was before the use of pesticide.[111]
Alternatives
While various measures for minimizing pesticide use apply to gardens,[112] they are not relevant to agriculture on scale.
Biological controls such as resistant plant varieties and the use of pheromones, have been successful and at times permanently resolve a pest problem.[113] Integrated Pest Management (IPM) employs chemical use only when other alternatives are ineffective. IPM causes less harm to humans and the environment. The focus is broader than on a specific pest, considering a range of pest control alternatives.[114] Biotechnology can also be an innovative way to control pests. Strains can be genetically modified (GM) to increase their resistance to pests.[113]
Prior or during the development of synthetic pesticides, many natural ones were identified including pyrethrum, rotenone, nicotine, sabadilla, and quassin.[115] Synthetic compounds proved cheaper and far more effective than natural pesticides.[116]
Biopesticides such as canola oil and baking soda that contain curtain active ingredients from natural substances are an environmentally friendly alternative for toxic pesticides.[117] There are three categories of biopesticides; microbial pesticides, plant-incorporated protectants (PIPs), and biochemical biopesticides. The alternatives to pesticides include a range of genetic material introduction to plants that target a particular pest, and active ingredients that control the mating and reproduction of certain pests or kill target pests.[117] Biopesticides are affective in small quantities and degrade quickly making them an eco-friendly alternative to pesticides.[118] They are often used in Integrated Pest Management (IPM) as well and has been an important component to the UK IPM strategy for its crop protection.[119]
Waste and disposal
In the United States, the Environmental Protection Agency (EPA) suggests proper use of pesticides and disposal that follows federal or individual state guidance for farmers or commercial users.[120] Commercial users of pesticides are told to follow the disposal instructions on the labels of the pesticides while using necessary safety measures for the disposal of hazardous waste.[120] They are also advised to call for assistance by their local agencies in the disposal of unwanted or unused pesticides.[120]
Still are there environmental problems that emerge from runoff and other negative effects of pesticides. Runoff of pesticides into wastewater and pesticide drift into other ecosystems has led to research in the removal and remediation of pesticides in the environment. Research has been done on different methods to treat pesticide pollution including the use of activated carbon absorption and advanced oxidation processes. Different methods of pesticide removal require different costs and can carry different removal outcomes. Some methods require low cost techniques but many result in byproducts that require an extra cost for removal or unwarranted environmental impacts.[121]
There is an ongoing research focused on pesticide removal, a 2022 study for example demonstrated excellent removal efficiency of 80% for often used pesticide chlorpyrifos through usage magnetic plant biobots.[122]
Activated carbon absorption
Due to the properties of activated carbon, different types have been researched as potential treatment for absorbing different pesticide species.[123] Researchers found a use for activated carbon from tangerine seeds in the absorption of pesticides.[124] Researches are utilizing this tangerine seed activated carbon in the removal process of carbamate pesticides that have been linked to an increased risk of cancer and other health risks.[124] Absorption by activated carbon has been found to be a successful and cost-efficient way of removing pesticides.[124]
Advanced oxidation process (AOP)
Advanced oxidation processes have been used to combat against the problem of pesticide residue on fruits and vegetables. AOP and its technologies have been used in the removal efforts of pesticide pollutants in wastewater using different chemical reactions to target different pollutants.[125] Researchers have found this method of pesticide removal using coupled free chlorine/ultrasound to be successful at removing pesticide residue from vegetables.[126]
Activism
Pesticide Action Network
While dubbed economic and ecologically sound practices by suppliers, the effects of agricultural pesticides can include toxicity, bioaccumulation, persistence, and physiological responses in humans and wildlife,[127] and several international NGOs, such as Pesticide Action Network, have risen in response to the economic activities of these larger transnational corporations. Historically, PAN's contributions targeting the Dirty Dozen have resulted in treaties and global environmental law banning persistent organic pollutants (POPs), such as endosulfan, and their campaign work on Prior Informed Consent (PIC) for countries in the Global South to know what hazardous and banned chemicals they might be importing have contributed to the culmination of the Rotterdam Convention on Prior Informed Consent, which went into effect in 2004.[128] PAN's work, according to their website, involves "shifting global aid away from pesticides",[129] in addition to community monitoring and serving as a watchdog for the World Bank policy failures.[129] Additionally, Pesticide Action Network members helped co-author the International Assessment of Agricultural Knowledge, Science and Technology for Development (IAASTD), working to center agroecological knowledge and farming techniques as crucial to the future of agriculture.[129]
See also
Further reading
- Tudi, Muyesaier; Daniel Ruan, Huada; Wang, Li; Lyu, Jia; Sadler, Ross; Connell, Des; Chu, Cordia; Phung, Dung Tri (January 2021). "Agriculture Development, Pesticide Application and Its Impact on the Environment". International Journal of Environmental Research and Public Health. 18 (3): 1112. doi:10.3390/ijerph18031112. ISSN 1660-4601. PMC 7908628. PMID 33513796.
- Rajak, Prem; Roy, Sumedha; Ganguly, Abhratanu; Mandi, Moutushi; Dutta, Anik; Das, Kanchana; Nanda, Sayantani; Ghanty, Siddhartha; Biswas, Gopal (May 2023). "Agricultural pesticides – friends or foes to biosphere?". Journal of Hazardous Materials Advances. 10. doi:10.1016/j.hazadv.2023.100264 – via Science Direct.
References
- ^ a b c George Tyler Miller (1 January 2004). Sustaining the Earth: An Integrated Approach. Thomson/Brooks/Cole. pp. 211–216. ISBN 978-0-534-40088-0.
- ^ Tashkent (1998), Part 75. Conditions and provisions for developing a national strategy for biodiversity conservation Archived 13 October 2007 at the Wayback Machine. Biodiversity Conservation National Strategy and Action Plan of Republic of Uzbekistan. Prepared by the National Biodiversity Strategy Project Steering Committee with the Financial Assistance of The Global Environmental Facility (GEF) and Technical Assistance of United Nations Development Programme (UNDP). Retrieved on 17 September 2007.
- ^ a b Damalas, C. A.; Eleftherohorinos, I. G. (2011). "Pesticide Exposure, Safety Issues, and Risk Assessment Indicators". International Journal of Environmental Research and Public Health. 8 (12): 1402–19. doi:10.3390/ijerph8051402. PMC 3108117. PMID 21655127.
- ^ "A third of global farmland at 'high' pesticide pollution risk". phys.org. Retrieved 22 April 2021.
- ^ Tang, Fiona H. M.; Lenzen, Manfred; McBratney, Alexander; Maggi, Federico (April 2021). "Risk of pesticide pollution at the global scale". Nature Geoscience. 14 (4): 206–210. Bibcode:2021NatGe..14..206T. doi:10.1038/s41561-021-00712-5. ISSN 1752-0908.
- ^ Lamberth, C.; Jeanmart, S.; Luksch, T.; Plant, A. (2013). "Current Challenges and Trends in the Discovery of Agrochemicals". Science. 341 (6147): 742–6. Bibcode:2013Sci...341..742L. doi:10.1126/science.1237227. PMID 23950530. S2CID 206548681.
- ^ Tosi, S.; Costa, C.; Vesco, U.; Quaglia, G.; Guido, G. (2018). "A survey of honey bee-collected pollen reveals widespread contamination by agricultural pesticides". The Science of the Total Environment. 615: 208–218. doi:10.1016/j.scitotenv.2017.09.226. PMID 28968582. S2CID 19956612.
- ^ a b "Pesticides and Pollinators". extension.psu.edu. Retrieved 1 May 2024.
- ^ Rajak, Prem; Roy, Sumedha; Ganguly, Abhratanu; Mandi, Moutushi; Dutta, Anik; Das, Kanchana; Nanda, Sayantani; Ghanty, Siddhartha; Biswas, Gopal (1 May 2023). "Agricultural pesticides – friends or foes to biosphere?". Journal of Hazardous Materials Advances. 10: 100264. doi:10.1016/j.hazadv.2023.100264. ISSN 2772-4166.
- ^ Tan, Sharlene. "What to Know About Natural Pesticides". WebMD. Retrieved 1 May 2024.
- ^ Andrew H. Cobb; John P. H. Reade (2011). "7.1". Herbicides and Plant Physiology. John Wiley & Sons. ISBN 9781444322491.
- ^ Troyer, James R. (March 2001). "In the beginning: the multiple discovery of the first hormone herbicides". Weed Science. 49 (2): 290–297. doi:10.1614/0043-1745(2001)049[0290:ITBTMD]2.0.CO;2. ISSN 0043-1745. S2CID 85637273.
- ^ Robert L Zimdahl (2007). A History of Weed Science in the United States. Elsevier. ISBN 9780123815026.
- ^ Quastel, J. H. (1950). "2,4-Dichlorophenoxyacetic Acid (2,4-D) as a Selective Herbicide". Agricultural Control Chemicals. Advances in Chemistry. Vol. 1. 1155 Sixteenth Street, N.W. Washington 6, D. C: American Chemical Society. pp. 244–249. doi:10.1021/ba-1950-0001.ch045. ISBN 978-0-8412-2442-1.
{{cite book}}
: CS1 maint: location (link) - ^ Metcalf Deceased, Robert L.; Horowitz, Abraham Rami (2014). "Insect Control, 2. Individual Insecticides". Ullmann's Encyclopedia of Industrial Chemistry. pp. 1–94. doi:10.1002/14356007.s14_s01. ISBN 9783527306732.
- ^ a b c d Kohler, H. -R.; Triebskorn, R. (2013). "Wildlife Ecotoxicology of Pesticides: Can We Track Effects to the Population Level and Beyond?". Science. 341 (6147): 759–765. Bibcode:2013Sci...341..759K. doi:10.1126/science.1237591. PMID 23950533. S2CID 206548843.
- ^ Pretty, Jules; Bharucha, Zareen (5 March 2015). "Integrated Pest Management for Sustainable Intensification of Agriculture in Asia and Africa". Insects. 6 (1): 152–182. doi:10.3390/insects6010152. ISSN 2075-4450. PMC 4553536. PMID 26463073.
- ^ "Ranking List of 2019 Top 20 Global Agrochemical Companies Recomposed, Graced by 11 Chinese Players". Grainews. Retrieved 28 April 2021.
- ^ Donley, Nathan (7 June 2019). "The USA lags behind other agricultural nations in banning harmful pesticides". Environmental Health. 18 (1): 44. Bibcode:2019EnvHe..18...44D. doi:10.1186/s12940-019-0488-0. ISSN 1476-069X. PMC 6555703. PMID 31170989.
- ^ EPA. 2011. Pesticides industry sales and usage; 2006 and 2007 market estimates. "Archived copy" (PDF). Archived from the original (PDF) on 18 March 2015. Retrieved 24 July 2014.
{{cite web}}
: CS1 maint: archived copy as title (link) - ^ USDA ERS. 2013. Table 1. Indices of farm output, input and total factor productivity for the United States, 1948–2011. (last update 27 September 2013) http://www.ers.usda.gov/data-products/agricultural-productivity-in-the-us.aspx#28247
- ^ a b c Turusov, V; Rakitsky, V; Tomatis, L (2002). "Dichlorodiphenyltrichloroethane (DDT): Ubiquity, persistence, and risks". Environmental Health Perspectives. 110 (2): 125–8. doi:10.1289/ehp.02110125. PMC 1240724. PMID 11836138.
- ^ a b c d e f g h Rattner, B. A. (2009). "History of wildlife toxicology". Ecotoxicology. 18 (7): 773–783. Bibcode:2009Ecotx..18..773R. doi:10.1007/s10646-009-0354-x. PMID 19533341. S2CID 23542210.
- ^ a b c d Fleischli, M. A.; Franson, J. C.; Thomas, N. J.; Finley, D. L.; Riley, W. (2004). "Avian Mortality Events in the United States Caused by Anticholinesterase Pesticides: A Retrospective Summary of National Wildlife Health Center Records from 1980 to 2000". Archives of Environmental Contamination and Toxicology. 46 (4): 542–50. Bibcode:2004ArECT..46..542F. CiteSeerX 10.1.1.464.4457. doi:10.1007/s00244-003-3065-y. PMID 15253053. S2CID 16852092.
- ^ Crain, D. A.; Guillette Jr, L. J. (1998). "Reptiles as models of contaminant-induced endocrine disruption". Animal Reproduction Science. 53 (1–4): 77–86. doi:10.1016/s0378-4320(98)00128-6. PMID 9835368.
- ^ a b c d e f Galloway, T. S.; Depledge, M. H. (2001). "Immunotoxicity in Invertebrates: Measurement and Ecotoxicological Relevance". Ecotoxicology. 10 (1): 5–23. doi:10.1023/A:1008939520263. PMID 11227817. S2CID 28285029.
- ^ Dzugan, S. A.; Rozakis, G. W.; Dzugan, K. S.; Emhof, L; Dzugan, S. S.; Xydas, C; Michaelides, C; Chene, J; Medvedovsky, M (2011). "Correction of steroidopenia as a new method of hypercholesterolemia treatment". Neuro Endocrinology Letters. 32 (1): 77–81. PMID 21407165.
- ^ a b c Galloway, T.; Handy, R. (2003). "Immunotoxicity of Organophosphorous Pesticides". Ecotoxicology. 12 (1–4): 345–363. doi:10.1023/A:1022579416322. PMID 12739880. S2CID 27561455.
- ^ a b Story, P.; Cox, M. (2001). "Review of the effects of organophosphorus and carbamate insecticides on vertebrates. Are there implications for locust management in Australia?". Wildlife Research. 28 (2): 179. doi:10.1071/WR99060.
- ^ Rohr, J. R.; Schotthoefer, A. M.; Raffel, T. R.; Carrick, H. J.; Halstead, N.; Hoverman, J. T.; Johnson, C. M.; Johnson, L. B.; Lieske, C.; Piwoni, M. D.; Schoff, P. K.; Beasley, V. R. (2008). "Agrochemicals increase trematode infections in a declining amphibian species". Nature. 455 (7217): 1235–1239. Bibcode:2008Natur.455.1235R. doi:10.1038/nature07281. PMID 18972018. S2CID 4361458.
- ^ Lin, P. C.; Lin, H. J.; Liao, Y. Y.; Guo, H. R.; Chen, K. T. (2013). "Acute poisoning with neonicotinoid insecticides: A case report and literature review". Basic & Clinical Pharmacology & Toxicology. 112 (4): 282–6. doi:10.1111/bcpt.12027. PMID 23078648. S2CID 3090396.
- ^ Gill, R. J.; Ramos-Rodriguez, O.; Raine, N. E. (2012). "Combined pesticide exposure severely affects individual- and colony-level traits in bees". Nature. 491 (7422): 105–108. Bibcode:2012Natur.491..105G. doi:10.1038/nature11585. PMC 3495159. PMID 23086150.
- ^ Henry, M.; Beguin, M.; Requier, F.; Rollin, O.; Odoux, J. -F.; Aupinel, P.; Aptel, J.; Tchamitchian, S.; Decourtye, A. (2012). "A Common Pesticide Decreases Foraging Success and Survival in Honey Bees" (PDF). Science. 336 (6079): 348–350. Bibcode:2012Sci...336..348H. doi:10.1126/science.1215039. PMID 22461498. S2CID 41186355.
- ^ Cresswell, J. E.; Thompson, H. M. (2012). "Comment on "A Common Pesticide Decreases Foraging Success and Survival in Honey Bees"". Science. 337 (6101): 1453. Bibcode:2012Sci...337.1453C. doi:10.1126/science.1224618. PMID 22997307.
- ^ Tosi, S.; Nieh, J. C. (10 April 2019). "Lethal and sublethal synergistic effects of a new systemic pesticide, flupyradifurone (Sivanto®), on honeybees". Proceedings of the Royal Society B: Biological Sciences. 286 (1900): 20190433. doi:10.1098/rspb.2019.0433. PMC 6501679. PMID 30966981.
- ^ Tong, Linda; Nieh, James C.; Tosi, Simone (1 December 2019). "Combined nutritional stress and a new systemic pesticide (flupyradifurone, Sivanto®) reduce bee survival, food consumption, flight success, and thermoregulation". Chemosphere. 237: 124408. Bibcode:2019Chmsp.23724408T. doi:10.1016/j.chemosphere.2019.124408. ISSN 0045-6535. PMID 31356997.
- ^ Pisa, Lennard; Goulson, Dave; Yang, En-Cheng; Gibbons, David; Sánchez-Bayo, Francisco; Mitchell, Edward; Aebi, Alexandre; van der Sluijs, Jeroen; MacQuarrie, Chris J. K.; Giorio, Chiara; Long, Elizabeth Yim (9 November 2017). "An update of the Worldwide Integrated Assessment (WIA) on systemic insecticides. Part 2: impacts on organisms and ecosystems". Environmental Science and Pollution Research. 28 (10): 11749–11797. doi:10.1007/s11356-017-0341-3. ISSN 1614-7499. PMC 7921077. PMID 29124633.
- ^ Biondi, A.; Mommaerts, V.; Smagghe, G.; Viñuela, E.; Zappalà, L.; Desneux, N. (2012). "The non-target impact of spinosyns on beneficial arthropods". Pest Management Science. 68 (12): 1523–1536. doi:10.1002/ps.3396. PMID 23109262.
- ^ a b Freemark, K. (1995). "Impacts of agricultural herbicide use on terrestrial wildlife in temperate landscapes: A review with special reference to North America". Agriculture, Ecosystems & Environment. 52 (2–3): 67–91. Bibcode:1995AgEE...52...67F. doi:10.1016/0167-8809(94)00534-L.
- ^ Cahill, J. F.; Elle, E.; Smith, G. R.; Shore, B. H. (2008). "Disruption of a Belowground Mutualism Alters Interactions Between Plants and Their Floral Visitors". Ecology. 89 (7): 1791–1801. Bibcode:2008Ecol...89.1791C. doi:10.1890/07-0719.1. PMID 18705367.
- ^ Newton, I. (2004). "The recent declines of farmland bird populations in Britain: An appraisal of causal factors and conservation actions". Ibis. 146 (4): 579–600. doi:10.1111/j.1474-919X.2004.00375.x.
- ^ a b Ritter L, Solomon KR, and Forget J, Stemeroff M, and O'Leary C. Persistent organic pollutants: An Assessment Report on: DDT, Aldrin, Dieldrin, Endrin, Chlordane, Heptachlor, Hexachlorobenzene, Mirex, Toxaphene, Polychlorinated Biphenyls, Dioxins and Furans Archived 26 September 2007 at the Wayback Machine. Prepared for The International Programme on Chemical Safety (IPCS), within the framework of the Inter-Organization Programme for the Sound Management of Chemicals (IOMC). Retrieved on 16 September 2007.
- ^ Centers for Disease Control and Prevention. Pesticides Archived 13 October 2007 at the Wayback Machine. cdc.gov. Retrieved on 15 September 2007.
- ^ Authority, Australian Pesticides and Veterinary Medicines (31 March 2015). "Tebufenozide in the product Mimic 700 WP Insecticide, Mimic 240 SC Insecticide". Australian Pesticides and Veterinary Medicines Authority.
- ^ Fantke, Peter; Gillespie, Brenda W.; Juraske, Ronnie; Jolliet, Olivier (2014). "Estimating Half-Lives for Pesticide Dissipation from Plants". Environmental Science & Technology. 48 (15): 8588–8602. Bibcode:2014EnST...48.8588F. doi:10.1021/es500434p. hdl:20.500.11850/91972. PMID 24968074.
- ^ npic.orst.edu: " Pesticide Half-life Fact Sheet", 2015
- ^ npic.orst.edu: "What Happens to Pesticides Released in the Environment?", 20 September 2017
- ^ usu.edu: "PESTICIDE ADSORPTION AND HALF-LIFE", October 2004
- ^ usu.edu: "PESTICIDE ADSORPTION AND HALF-LIFE", February 1999
- ^ a b c d e Cornell University. Pesticides in the environment Archived 5 June 2009 at the Wayback Machine. Pesticide fact sheets and tutorial, . Pesticide Safety Education Program. Retrieved on 11 October 2007.
- ^ National Park Service. US Department of the Interior. (1 August 2006), Sequoia & Kings Canyon National Park: Air quality – Airborne synthetic chemicals. Nps.gov. Retrieved on 19 September 2007.
- ^ "PRN 2001-X Draft: Spray and Dust Drift Label Statements for Pesticide Products". Pesticide Registration. Washington, DC: US Environmental Protection Agency (EPA). Retrieved 19 September 2007.
- ^ Environment Canada (September–October 2001), Agricultural pesticides and the atmosphere Archived 24 September 2006 at the Wayback Machine. Retrieved on 12 October 2007.
- ^ a b c d e Palmer, WE, Bromley, PT, and Brandenburg, RL. Wildlife & pesticides – Peanuts. North Carolina Cooperative Extension Service. Retrieved on 11 October 2007.
- ^ a b Science Daily (19 November 1999), Evergreens help block spread of pesticide from crop fields. Sciencedaily.com. Retrieved on 19 September 2007.
- ^ UC IPM Online. (11 August 2006), What's up, Doc? Maybe less air pollution. Statewide IPM Program, Agriculture and Natural Resources, University of California. Ipm.ucdavis.edu. Retrieved on 15 October 2007.
- ^ Gillion, RJ; Barbash, JE; Crawford, GG; Hamilton, PA; Martin, JD; Nakagaki, N; Nowell, LH; Scott, JC; Stackelberg, PE; Thelin, GP; Wolock, DM (15 February 2007) [2006]. "1. Overview of Findings and Implications". Pesticides in the Nation's Streams and Ground Water, 1992–2001 (Report). The Quality of Our Nation's Waters. Reston, VA: US Geological Survey. p. 4. Circular 1291.
- ^ a b c d Kellogg RL, Nehring R, Grube A, Goss DW, and Plotkin S (February 2000), Environmental indicators of pesticide leaching and runoff from farm fields Archived 18 June 2002 at the Wayback Machine. United States Department of Agriculture Natural Resources Conservation Service. Retrieved on 3 October 2007.
- ^ Bingham, S (2007), Pesticides in rivers and groundwater Archived 2 March 2009 at the Wayback Machine. Environment Agency, UK. Retrieved on 12 October 2007.
- ^ Hogan,, CM, Patmore L, Latshaw, G, Seidman, H, et al. (1973), Computer modeling of pesticide transport in soil for five instrumented watersheds, U.S. Environmental Protection Agency Southeast Water laboratory, Athens, Ga. by ESL Inc., Sunnyvale, California.
- ^ States of Jersey (2007), Environmental protection and pesticide use Archived 25 August 2006 at the Wayback Machine. Retrieved on 10 October 2007.
- ^ Papendick, RI; Elliott, LF; Dahlgren, RB (1986). "Environmental consequences of modern production agriculture: How can alternative agriculture address these issues and concerns?". American Journal of Alternative Agriculture. 1 (1): 3–10. doi:10.1017/s0889189300000722.
- ^ a b Pedersen, TL (June 1997), Pesticide residues in drinking water. extoxnet.orst.edu. Retrieved on 15 September 2007.
- ^ "Private Drinking Water Wells". EPA. 15 November 2016.
- ^ "How Are Water Quality Standards Developed?". Standards for Water Body Health. EPA. 3 November 2016.
- ^ "State-Specific Water Quality Standards Effective under the Clean Water Act (CWA)". EPA. 1 December 2016.
- ^ a b Bingham, S (2007), Pesticides exceeding environmental quality standards (EQS) Archived 17 June 2008 at the Wayback Machine. The Environment Agency, UK. Retrieved on 12 October 2007.
- ^ Environmental, Oakshire. "How To Test For Agricultural Land Contamination". Oakshire Environmental. Retrieved 23 January 2020.
- ^ a b Hussain S, Siddique T, Saleem M, Arshad M, Khalid A (2009). Chapter 5: Impact of Pesticides on Soil Microbial Diversity, Enzymes, and Biochemical Reactions. Advances in Agronomy. Vol. 102. pp. 159–200. doi:10.1016/s0065-2113(09)01005-0. ISBN 9780123748188.
- ^ Abdel-Mallek AY, Moharram AM, Abdel-Kader MI, Omar SA (1994). "Effect of soil treatment with the organophosphorus insecticide Profenfos on the fungal flora and some microbial activities". Microbiological Research. 149 (2): 167–171. doi:10.1016/s0944-5013(11)80114-x. PMID 7921896.
- ^ "Sources of common contaminants and their health effects". Emergency Response Program. EPA. Archived from the original on 20 December 2008. Retrieved 10 October 2007.
- ^ Johnston, AE (1986). "Soil organic-matter, effects on soils and crops". Soil Use Management. 2 (3): 97–105. Bibcode:1986SUMan...2...97J. doi:10.1111/j.1475-2743.1986.tb00690.x.
- ^ Lotter DW, Seidel R, Liebhardt W (2003). "The performance of organic and conventional cropping systems in an extreme climate year". American Journal of Alternative Agriculture. 18 (3): 146–154. doi:10.1079/AJAA200345.
- ^ Arias-Estévez, Manuel; Eugenio López-Periago; Elena Martínez-Carballo; Jesús Simal-Gándara; Juan-Carlos Mejuto; Luis García-Río (February 2008). "The mobility and degradation of pesticides in soils and the pollution of groundwater resources" (PDF). Agriculture, Ecosystems & Environment. 123 (4): 247–260. Bibcode:2008AgEE..123..247A. doi:10.1016/j.agee.2007.07.011. ISSN 0167-8809. Archived from the original (PDF) on 25 April 2012. Retrieved 10 November 2011.
- ^ a b c Rockets, Rusty (8 June 2007), Down On The Farm? Yields, Nutrients And Soil Quality. Scienceagogo.com. Retrieved on 15 September 2007.
- ^ Fox, JE; Gulledge, J; Engelhaupt, E; Burrow, ME & McLachlan, JA (2007). "Pesticides reduce symbiotic efficiency of nitrogen-fixing rhizobia and host plants". Proceedings of the National Academy of Sciences of the USA. 104 (24): 10282–10287. Bibcode:2007PNAS..10410282F. doi:10.1073/pnas.0611710104. PMC 1885820. PMID 17548832.
- ^ Walley F, Taylor A and Lupwayi (2006) Herbicide effects on pulse crop nodulation and nitrogen fixation. FarmTech 2006 Proceedings 121–123.
- ^ Dicks, Lynn V.; Breeze, Tom D.; Ngo, Hien T.; Senapathi, Deepa; An, Jiandong; Aizen, Marcelo A.; Basu, Parthiba; Buchori, Damayanti; Galetto, Leonardo; Garibaldi, Lucas A.; Gemmill-Herren, Barbara; Howlett, Brad G.; Imperatriz-Fonseca, Vera L.; Johnson, Steven D.; Kovács-Hostyánszki, Anikó; Kwon, Yong Jung; Lattorff, H. Michael G.; Lungharwo, Thingreipi; Seymour, Colleen L.; Vanbergen, Adam J.; Potts, Simon G. (16 August 2021). "A global-scale expert assessment of drivers and risks associated with pollinator decline". Nature Ecology & Evolution. 5 (10): 1453–1461. Bibcode:2021NatEE...5.1453D. doi:10.1038/s41559-021-01534-9. PMID 34400826. S2CID 237148742.
- ^ Hackenberg D (14 March 2007). "Letter from David Hackenberg to American growers from March 14, 2007". Plattform Imkerinnen – Austria. Archived from the original on 14 June 2007. Retrieved 27 March 2007.
- ^ "Pesticides and Pollinators". extension.psu.edu. Retrieved 29 April 2024.
- ^ Wells, M (11 March 2007). "Vanishing bees threaten US". www.bbc.co.uk. BBC News. Retrieved 19 September 2007.
- ^ Haefeker, Walter (12 August 2000). "Betrayed and sold out – German bee monitoring". Retrieved 10 October 2007.
- ^ Zeissloff, Eric (2001). "Schadet imidacloprid den bienen" (in German). Retrieved 10 October 2007.
- ^ Yasmin, S.; d'Souza, D. (2010). "Effects of Pesticides on the Growth and Reproduction of Earthworm: A Review". Applied and Environmental Soil Science. 2010: 1–9. doi:10.1155/2010/678360.
- ^ a b Duval, Guillaume (11 April 2018). "Birds – collateral victims of intensive agriculture". Alternatives Economiques/EDJNet. Retrieved 24 August 2018.
- ^ Fimrite, Peter (27 June 2011). "Suit says EPA fails to shield species from poisons". The San Francisco Chronicle.
- ^ Sauer, John R.; Link, William A.; Hines, James E. (2020). "Wildlife Biology". The North American Breeding Bird Survey, Analysis Results 1966 - 2019 - ScienceBase-Catalog. U.S. Geological Survey. doi:10.5066/p96a7675.
- ^ Kerbs JR, Wilson JD, Bradbury RB, and Siriwardena GM (12 August 1999), The second silent spring Archived 6 April 2008 at the Wayback Machine. Commentary in Nature, Volume 400, Pages 611–612.
- ^ a b Vos, J. G.; Dybing, E; Greim, H. A.; Ladefoged, O; Lambré, C; Tarazona, J. V.; Brandt, I; Vethaak, A. D. (2000). "Health effects of endocrine-disrupting chemicals on wildlife, with special reference to the European situation". Critical Reviews in Toxicology. 30 (1): 71–133. doi:10.1080/10408440091159176. PMID 10680769. S2CID 11908661.
- ^ a b c d e Helfrich, LA, Weigmann, DL, Hipkins, P, and Stinson, ER (June 1996), Pesticides and aquatic animals: A guide to reducing impacts on aquatic systems Archived 5 March 2009 at the Wayback Machine. Virginia Cooperative Extension. Retrieved on 14 October 2007.
- ^ Toughill K (1999), The summer the rivers died: Toxic runoff from potato farms is poisoning P.E.I. Archived 18 January 2008 at the Wayback Machine Originally published in Toronto Star Atlantic Canada Bureau. Retrieved on 17 September 2007.
- ^ Pesticide Action Network North America (4 June 1999), Pesticides threaten birds and fish in California Archived 18 February 2012 at the Wayback Machine. PANUPS. Retrieved on 17 September 2007.
- ^ Cone M (6 December 2000), A wind-borne threat to Sierra frogs: A study finds that pesticides used on farms in the San Joaquin Valley damage the nervous systems of amphibians in Yosemite and elsewhere Archived 2 November 2015 at the Wayback Machine. L.A. Times Retrieved on 17 September 2007.
- ^ a b Science Daily (3 February 2006), Pesticide combinations imperil frogs, probably contribute to amphibian decline. Sciencedaily.com. Retrieved on 16 October 2007.
- ^ Raloff, J (5 September 1998) Common pesticide clobbers amphibians. Science News, Volume 154, Number 10, Page 150. Retrieved on 15 October 2007.
- ^ a b California Department of Pesticide Regulation (2008), "What are the Potential Health Effects of Pesticides?" Community Guide to Recognizing and Reporting Pesticide Problems. Sacramento, CA. Pages 27–29.
- ^ a b Lorenz, Eric S. (2009). "Potential Health Effects of Pesticides" (PDF). Ag Communications and Marketing: 1–8. Archived from the original (PDF) on 11 August 2013. Retrieved 1 February 2014.
- ^ Du Toit, D. F. (1992). "Pancreatic transplantation". South African Medical. 81 (8): 432–3. PMID 1566222.
- ^ Mie, Axel; Andersen, Helle Raun; Gunnarsson, Stefan; Kahl, Johannes; Kesse-Guyot, Emmanuelle; Rembiałkowska, Ewa; Quaglio, Gianluca; Grandjean, Philippe (27 October 2017). "Human health implications of organic food and organic agriculture: a comprehensive review". Environmental Health. 16 (1): 111. Bibcode:2017EnvHe..16..111M. doi:10.1186/s12940-017-0315-4. ISSN 1476-069X. PMC 5658984. PMID 29073935.
- ^ Crawford, S. L.; Fiedler, E. R. (1992). "Childhood physical and sexual abuse and failure to complete military basic training". Military Medicine. 157 (12): 645–8. doi:10.1093/milmed/157.12.645. PMID 1470375.
- ^ Hodgson, E; Levi, P. E. (1996). "Pesticides: An important but underused model for the environmental health sciences". Environmental Health Perspectives. 104 (Suppl 1): 97–106. doi:10.1289/ehp.96104s197. PMC 1469573. PMID 8722114.
- ^ a b PBS (2001), Pesticide resistance. Retrieved on September 15, 2007.
- ^ Guedes, R.N.C.; Smagghe, G.; Stark, J.D.; Desneux, N. (11 March 2016). "Pesticide-Induced Stress in Arthropod Pests for Optimized Integrated Pest Management Programs". Annual Review of Entomology. 61 (1). Annual Reviews: 43–62. doi:10.1146/annurev-ento-010715-023646. ISSN 0066-4170. PMID 26473315. S2CID 207747295.
- ^ "Resistance Definition". Insecticide Resistance Action Committee. 2007.
- ^ Grapes at Missouri State University (MSU) How pesticide resistance develops Archived 2007-08-17 at the Wayback Machine. Excerpt from: Larry Gut, Annemiek Schilder, Rufus Isaacs and Patricia McManus. Fruit Crop Ecology and Management, Chapter 2: "Managing the Community of Pests and Beneficials." Retrieved on September 15, 2007.
- ^ Miller GT (2004), Sustaining the Earth, 6th edition. Thompson Learning, Inc. Pacific Grove, California. Chapter 9, Pages 211-216.
- ^ Levine, E; Oloumi-Sadeghi, H; Fisher, JR (1992). "Discovery of multiyear diapause in Illinois and South Dakota Northern corn rootworm (Coleoptera: Cerambycidae) eggs and incidence of the prolonged diapause trait in Illinois". Journal of Economic Entomology. 85: 262–267. doi:10.1093/jee/85.1.262.
- ^ Service, Robert F. (20 September 2013). "What Happens When Weed Killers Stop Killing?". Science. 341 (6152): 1329. doi:10.1126/science.341.6152.1329. PMID 24052282.
- ^ Guedes, R. N. C.; Roditakis, E.; Campos, M. R.; Haddi, K.; Bielza, P.; Siqueira, H. A. A.; Tsagkarakou, A.; Vontas, J.; Nauen, R. (31 January 2019). "Insecticide resistance in the tomato pinworm Tuta absoluta: patterns, spread, mechanisms, management and outlook". Journal of Pest Science. 92 (4). Springer: 1329–1342. doi:10.1007/s10340-019-01086-9. ISSN 1612-4758. S2CID 59524736.
- ^ Muckenfuss AE, Shepard BM, Ferrer ER, Natural mortality of diamondback moth in coastal South Carolina Archived 15 February 2012 at the Wayback Machine Clemson University, Coastal Research and Education Center.
- ^ a b c Howell V. Daly; John T. Doyen; Alexander H. Purcell (1 January 1998). Introduction to Insect Biology and Diversity. Oxford University Press. pp. 279–300. ISBN 978-0-19-510033-4.
- ^ "Take Action! How to Eliminate Pesticide Use." (2003) National Audubon Society. Pages 1–3.
- ^ a b Lewis, W. J., J. C. van Lenteren, Sharad C. Phatak, and J. H. Tumlinson, III. "A total system approach to sustainable pest management." The National Academy of Sciences 13 August 1997. Web of Science.
- ^ Thad Godish (2 November 2000). Indoor Environmental Quality. CRC Press. pp. 325–326. ISBN 978-1-4200-5674-7.
- ^ Arnason, J. T.; Philogène, B. J. R.; Morand, Peter, eds. (23 February 1989). "Botanical Pesticides". Insecticides of Plant Origin. ACS Symposium Series. Vol. 387. Washington, DC: American Chemical Society. pp. 1–10. doi:10.1021/bk-1989-0387.ch001. ISBN 978-0-8412-1569-6.
- ^ Cheryl Wilen. "Natural Herbicides: Are they effective?".
- ^ a b US EPA, OCSPP (31 August 2015). "What are Biopesticides?". www.epa.gov. Retrieved 22 August 2022.
- ^ Sharma, Akanksha; Shukla, Ananya; Attri, Kriti; Kumar, Megha; Kumar, Puneet; Suttee, Ashish; Singh, Gurpal; Barnwal, Ravi Pratap; Singla, Neha (15 September 2020). "Global trends in pesticides: A looming threat and viable alternatives". Ecotoxicology and Environmental Safety. 201: 110812. Bibcode:2020EcoES.20110812S. doi:10.1016/j.ecoenv.2020.110812. ISSN 0147-6513. PMID 32512419. S2CID 219549853.
- ^ Chandler, David; Bailey, Alastair S.; Tatchell, G. Mark; Davidson, Gill; Greaves, Justin; Grant, Wyn P. (12 July 2011). "The development, regulation and use of biopesticides for integrated pest management". Philosophical Transactions of the Royal Society B: Biological Sciences. 366 (1573): 1987–1998. doi:10.1098/rstb.2010.0390. ISSN 0962-8436. PMC 3130386. PMID 21624919.
- ^ a b c US EPA, OCSPP (22 May 2015). "Requirements for Pesticide Disposal". www.epa.gov. Retrieved 22 August 2022.
- ^ Saleh, Iman A.; Zouari, Nabil; Al-Ghouti, Mohammad A. (1 August 2020). "Removal of pesticides from water and wastewater: Chemical, physical and biological treatment approaches". Environmental Technology & Innovation. 19: 101026. Bibcode:2020EnvTI..1901026S. doi:10.1016/j.eti.2020.101026. ISSN 2352-1864. S2CID 225364636.
- ^ Chemistry, University of; Prague, Technology. "Magnetic plant biobots can be effectively used for pesticide and heavy metal removal". phys.org. Retrieved 24 October 2022.
- ^ Foo, K. Y.; Hameed, B. H. (15 March 2010). "Detoxification of pesticide waste via activated carbon adsorption process". Journal of Hazardous Materials. 175 (1): 1–11. doi:10.1016/j.jhazmat.2009.10.014. ISSN 0304-3894. PMID 19879688.
- ^ a b c Wang, Yue; Wang, Shu-ling; Xie, Tian; Cao, Jun (1 November 2020). "Activated carbon derived from waste tangerine seed for the high-performance adsorption of carbamate pesticides from water and plant". Bioresource Technology. 316: 123929. Bibcode:2020BiTec.31623929W. doi:10.1016/j.biortech.2020.123929. ISSN 0960-8524. PMID 32763805. S2CID 221074896.
- ^ Comninellis, Christos; Kapalka, Agnieszka; Malato, Sixto; Parsons, Simon A; Poulios, Ioannis; Mantzavinos, Dionissios (June 2008). "Advanced oxidation processes for water treatment: advances and trends for R&D". Journal of Chemical Technology & Biotechnology. 83 (6): 769–776. Bibcode:2008JCTB...83..769C. doi:10.1002/jctb.1873.
- ^ Yang, Laxiang; Zhou, Jieqiong; Feng, Yuxin (23 December 2021). "Removal of pesticide residues from fresh vegetables by the coupled free chlorine/ultrasound process". Ultrasonics Sonochemistry. 82: 105891. doi:10.1016/j.ultsonch.2021.105891. ISSN 1350-4177. PMC 8799609. PMID 34954630.
- ^ David J. Hoffman; Barnett A. Rattner; G. Allen Burton Jr; John Cairns Jr, eds. (2003). Handbook of ecotoxicology (2nd ed.). Boca Raton: Lewis Publishers. ISBN 1-56670-546-0. OCLC 49952447.
- ^ Barrios, Paula (2003). The Rotterdam convention on hazardous chemicals and pesticides: a meaningful step towards environmental protection? (Master of Laws thesis). doi:10.14288/1.0077646.
- ^ a b c "Milestones | Pesticide Action Network". www.panna.org. 24 March 2014. Retrieved 2 August 2021.
External links
- National Pesticide Information Center – What happens to pesticides released in the environment?
- Streaming online video about efforts to reduce pesticide use in rice in Bangladesh. Windows Media Player [1], RealPlayer [2]
- Reptile Amphibian & Pesticide (RAP) Database
- EXtension TOXicology NETwork (Extoxnet) – pesticide information profiles. Environmental and health information broken down by type of pesticide