Gravitropism
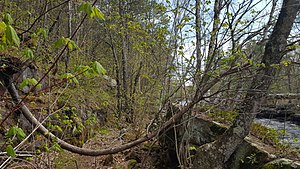

Gravitropism (also known as geotropism) is a coordinated process of differential growth by a plant in response to gravity pulling on it. It also occurs in fungi. Gravity can be either "artificial gravity"[clarification needed] or natural gravity. It is a general feature of all higher and many lower plants as well as other organisms. Charles Darwin was one of the first to scientifically document that roots show positive gravitropism and stems show negative gravitropism.[1] That is, roots grow in the direction of gravitational pull (i.e., downward) and stems grow in the opposite direction (i.e., upwards). This behavior can be easily demonstrated with any potted plant. When laid onto its side, the growing parts of the stem begin to display negative gravitropism, growing (biologists say, turning; see tropism) upwards. Herbaceous (non-woody) stems are capable of a degree of actual bending, but most of the redirected movement occurs as a consequence of root or stem growth outside.[clarification needed] The mechanism is based on the Cholodny–Went model which was proposed in 1927, and has since been modified.[2] Although the model has been criticized and continues to be refined, it has largely stood the test of time.[citation needed]
In roots

Root growth occurs by division of stem cells in the root meristem located in the tip of the root, and the subsequent asymmetric expansion of cells in a shoot-ward region to the tip known as the elongation zone. Differential growth during tropisms mainly involves changes in cell expansion versus changes in cell division, although a role for cell division in tropic growth has not been formally ruled out. Gravity is sensed in the root tip and this information must then be relayed to the elongation zone so as to maintain growth direction and mount effective growth responses to changes in orientation to and continue to grow its roots in the same direction as gravity.[4]
Abundant evidence demonstrates that roots bend in response to gravity due to a regulated movement of the plant hormone auxin known as polar auxin transport.[5] This was described in the 1920s in the Cholodny-Went model. The model was independently proposed by the Ukrainian scientist N. Cholodny of the University of Kyiv in 1927 and by Frits Went of the California Institute of Technology in 1928, both based on work they had done in 1926.[6] Auxin exists in nearly every organ and tissue of a plant, but it has been reoriented in the gravity field, can initiate differential growth resulting in root curvature.
Experiments show that auxin distribution is characterized by a fast movement of auxin to the lower side of the root in response to a gravity stimulus at a 90° degree angle or more. However, once the root tip reaches a 40° angle to the horizontal of the stimulus, auxin distribution quickly shifts to a more symmetrical arrangement. This behavior is described as a "tipping point" mechanism for auxin transport in response to a gravitational stimulus.[3]
In shoots

Gravitropism is an integral part of plant growth, orienting its position to maximize contact with sunlight, as well as ensuring that the roots are growing in the correct direction. Growth due to gravitropism is mediated by changes in concentration of the plant hormone auxin within plant cells.

As plants mature, gravitropism continues to guide growth and development along with phototropism. While amyloplasts continue to guide plants in the right direction, plant organs and function rely on phototropic responses to ensure that the leaves are receiving enough light to perform basic functions such as photosynthesis. In complete darkness, mature plants have little to no sense of gravity, unlike seedlings that can still orient themselves to have the shoots grow upward until light is reached when development can begin.[8]
Differential sensitivity to auxin helps explain Darwin's original observation that stems and roots respond in the opposite way to the forces of gravity. In both roots and stems, auxin accumulates towards the gravity vector on the lower side. In roots, this results in the inhibition of cell expansion on the lower side and the concomitant curvature of the roots towards gravity (positive gravitropism).[4][9] In stems, the auxin also accumulates on the lower side, however in this tissue it increases cell expansion and results in the shoot curving up (negative gravitropism).[10]
A recent study showed that for gravitropism to occur in shoots, a lot of an inclination, instead of a weak gravitational force, is necessary. This finding sets aside gravity sensing mechanisms that would rely on detecting the pressure of the weight of statoliths.[11]
In fruit
A few species of fruit exhibit negative geotropism. Bananas are one well-known example.[12] Once the canopy that covers the fruit dries, the bananas will begin to curve upwards, towards sunlight, in what is known as phototropism. The specific chemical that initiates the upward curvature is a phytohormone in the banana called Auxin. When the banana is first exposed to sunlight after the leaf canopy dries, one face of the fruit is shaded. On exposure to sunlight, auxin in the banana migrates from the sunlight side to the shaded side. Since auxin is a powerful plant growth hormone, the increased concentration promotes cell division and causes the plant cells on the shaded side to grow.[13] This asymmetrical distribution of auxin is responsible for the upward curvature of the banana.[13][14]
Gravity-sensing mechanisms
Statoliths

Plants possess the ability to sense gravity in several ways, one of which is through statoliths. Statoliths are dense amyloplasts, organelles that synthesize and store starch involved in the perception of gravity by the plant (gravitropism), that collect in specialized cells called statocytes. Statocytes are located in the starch parenchyma cells near vascular tissues in the shoots and in the columella in the caps of the roots.[15] These specialized amyloplasts are denser than the cytoplasm and can sediment according to the gravity vector. The statoliths are enmeshed in a web of actin and it is thought that their sedimentation transmits the gravitropic signal by activating mechanosensitive channels.[4] The gravitropic signal then leads to the reorientation of auxin efflux carriers and subsequent redistribution of auxin streams in the root cap and root as a whole.[16] Auxin moves toward higher concentrations on the bottom side of the root and suppresses elongation. The asymmetric distribution of auxin leads to differential growth of the root tissues, causing the root to curve and follow the gravity stimuli. Statoliths are also found in the endodermic layer of the hypocotyl, stem, and inflorescence stock. The redistribution of auxin causes increased growth on the lower side of the shoot so that it orients in a direction opposite that of the gravity stimuli.
Modulation by phytochrome
Phytochromes are red and far-red photoreceptors that help induce changes in certain aspects of plant development. Apart being itself the tropic factor (phototropism), light may also suppress the gravitropic reaction.[17] In seedlings, red and far-red light both inhibit negative gravitropism in seedling hypocotyls (the shoot area below the cotyledons) causing growth in random directions. However, the hypocotyls readily orient towards blue light. This process may be caused by phytochrome disrupting the formation of starch-filled endodermal amyloplasts and stimulating their conversion to other plastid types, such as chloroplasts or etiolaplasts.[17]
Compensation

Bending mushroom stems follow some regularities that are not common in plants. After turning into horizontal the normal vertical orientation the apical part (region C in the figure below) starts to straighten. Finally this part gets straight again, and the curvature concentrates near the base of the mushroom.[18] This effect is called compensation (or sometimes, autotropism). The exact reason of such behavior is unclear, and at least two hypotheses exist.
- The hypothesis of plagiogravitropic reaction supposes some mechanism that sets the optimal orientation angle other than 90 degrees (vertical). The actual optimal angle is a multi-parameter function, depending on time, the current reorientation angle and from the distance to the base of the fungi. The mathematical model, written following this suggestion, can simulate bending from the horizontal into vertical position but fails to imitate realistic behavior when bending from the arbitrary reorientation angle (with unchanged model parameters).[18]
- The alternative model supposes some “straightening signal”, proportional to the local curvature. When the tip angle approaches 30° this signal overcomes the bending signal, caused by reorientation, straightening resulting.[19]
Both models fit the initial data well, but the latter was also able to predict bending from various reorientation angles. Compensation is less obvious in plants, but in some cases it can be observed combining exact measurements with mathematical models. The more sensitive roots are stimulated by lower levels of auxin; higher levels of auxin in lower halves stimulate less growth, resulting in downward curvature (positive gravitropism).
Gravitropic mutants
Mutants with altered responses to gravity have been isolated in several plant species including Arabidopsis thaliana (one of the genetic model systems used for plant research). These mutants have alterations in either negative gravitropism in hypocotyls and/or shoots, or positive gravitropism in roots, or both.[10] Mutants have been identified with varying effects on the gravitropic responses in each organ, including mutants which nearly eliminate gravitropic growth, and those whose effects are weak or conditional. In the same way that gravity has an effect on winding and circumnutating, thus aspects of morphogenesis have defects on the mutant. Once a mutant has been identified, it can be studied to determine the nature of the defect (the particular difference(s) it has compared to the non-mutant 'wildtype'). This can provide information about the function of the altered gene, and often about the process under study. In addition the mutated gene can be identified, and thus something about its function inferred from the mutant phenotype.
Gravitropic mutants have been identified that affect starch accumulation, such as those affecting the PGM1 (which encodes the enzyme phosphoglucomutase) gene in Arabidopsis, causing plastids – the presumptive statoliths – to be less dense and, in support of the starch-statolith hypothesis, less sensitive to gravity.[20] Other examples of gravitropic mutants include those affecting the transport or response to the hormone auxin.[10] In addition to the information about gravitropism which such auxin-transport or auxin-response mutants provide, they have been instrumental in identifying the mechanisms governing the transport and cellular action of auxin as well as its effects on growth.
There are also several cultivated plants that display altered gravitropism compared to other species or to other varieties within their own species. Some are trees that have a weeping or pendulate growth habit; the branches still respond to gravity, but with a positive response, rather than the normal negative response. Others are the lazy (i.e. ageotropic or agravitropic) varieties of corn (Zea mays) and varieties of rice, barley and tomatoes, whose shoots grow along the ground.
See also
- Amyloplast – starch organelle involved in sensing gravitropism
- Astrobotany – the field of science concerned with plants in a spaceflight environment
- Clinostat – a device used to the effects of gravitational pull
- Random positioning machine – a device used to negate the effects of gravitational pull
- Free fall machine – a device used to negate the effects of gravitational pull
- Large diameter centrifuge – a device used to create a hyper-gravity pull
- Prolonged sine – reaction of plants to turning from their usual vertical orientation
References
- ^ Darwin, Charles; Darwin, Francisc (1881). The power of movement in plants. New York: D. Appleton and Company. Retrieved 24 April 2018.
- ^ Haga, Ken; Takano, Makoto; Neumann, Ralf; Iino, Moritoshi (January 1, 2005). "The Rice Coleoptile Phototropisim1 Gene Encoding an Ortholog of Arabidopsis NPH3 Is Required for Phototropism of Coleoptiles and Lateral Translocation of Auxin(W)". Plant Cell. 17 (1): 103–15. doi:10.1105/tpc.104.028357. PMC 544493. PMID 15598797.
- ^ a b Band, L. R.; Wells, D. M.; Larrieu, A.; Sun, J.; Middleton, A. M.; French, A. P.; Brunoud, G.; Sato, E. M.; Wilson, M. H.; Peret, B.; Oliva, M.; Swarup, R.; Sairanen, I.; Parry, G.; Ljung, K.; Beeckman, T.; Garibaldi, J. M.; Estelle, M.; Owen, M. R.; Vissenberg, K.; Hodgman, T. C.; Pridmore, T. P.; King, J. R.; Vernoux, T.; Bennett, M. J. (5 March 2012). "Root gravitropism is regulated by a transient lateral auxin gradient controlled by a tipping-point mechanism". Proceedings of the National Academy of Sciences. 109 (12): 4668–4673. Bibcode:2012PNAS..109.4668B. doi:10.1073/pnas.1201498109. PMC 3311388. PMID 22393022.
- ^ a b c Perrin, Robyn M.; Young, Li-Sen; Narayana Murthy, U.M.; Harrison, Benjamin R.; Wang, Yan; WILL, Jessica L.; Masson, Patrick H. (2017-04-21). "Gravity Signal Transduction in Primary Roots". Annals of Botany. 96 (5): 737–743. doi:10.1093/aob/mci227. ISSN 0305-7364. PMC 4247041. PMID 16033778.
- ^ Swarup, Ranjan; Kramer, Eric M.; Perry, Paula; Knox, Kirsten; Leyser, H. M. Ottoline; Haseloff, Jim; Beemster, Gerrit T. S.; Bhalerao, Rishikesh; Bennett, Malcolm J. (2005-11-01). "Root gravitropism requires lateral root cap and epidermal cells for transport and response to a mobile auxin signal". Nature Cell Biology. 7 (11): 1057–1065. doi:10.1038/ncb1316. ISSN 1465-7392. PMID 16244669. S2CID 22337800.
- ^ Janick, Jules (2010). Horticultural Reviews. John Wiley & Sons. p. 235. ISBN 978-0-470-65053-0.
- ^ "Gravitropism Lesson". herbarium.desu.edu. Retrieved 2018-07-08.
- ^ Hangarter, R.P. (1997). "Gravity, light, and plant form". Plant, Cell & Environment. 20 (6): 796–800. doi:10.1046/j.1365-3040.1997.d01-124.x. PMID 11541207.
- ^ Hou, G., Kramer, V. L., Wang, Y.-S., Chen, R., Perbal, G., Gilroy, S. and Blancaflor, E. B. (2004). "The promotion of gravitropism in Arabidopsis roots upon actin disruption is coupled with the extended alkalinization of the columella cytoplasm and a persistent lateral auxin gradient". The Plant Journal. 39 (1): 113–125. doi:10.1111/j.1365-313x.2004.02114.x. PMID 15200646.
{{cite journal}}
: CS1 maint: multiple names: authors list (link) - ^ a b c Masson, Patrick H.; Tasaka, Masao; Morita, Miyo T.; Guan, Changhui; Chen, Rujin; Boonsirichai, Kanokporn (2002-01-01). "Arabidopsis thaliana: A Model for the Study of Root and Shoot Gravitropism". The Arabidopsis Book. 1: e0043. doi:10.1199/tab.0043. PMC 3243349. PMID 22303208.
- ^ Chauvet, Hugo; Pouliquen, Olivier; Forterre, Yoël; Legué, Valérie; Moulia, Bruno (14 October 2016). "Inclination not force is sensed by plants during shoot gravitropism". Scientific Reports. 6 (1): 35431. Bibcode:2016NatSR...635431C. doi:10.1038/srep35431. PMC 5064399. PMID 27739470.
- ^ Hu, Wei; Zuo, Jiao; Hou, Xiaowan; Yan, Yan; Wei, Yunxie; Liu, Juhua; Li, Meiying; Xu, Biyu; Jin, Zhiqiang (2015-09-15). "The auxin response factor gene family in banana: genome-wide identification and expression analyses during development, ripening, and abiotic stress". Frontiers in Plant Science. 6: 742. doi:10.3389/fpls.2015.00742. ISSN 1664-462X. PMC 4569978. PMID 26442055.
- ^ a b Strohm, A.; Baldwin, K.; Masson, P. H. (2013), "Gravitropism in Arabidopsis thaliana", in Maloy, Stanley; Hughes, Kelly (eds.), Brenner's Encyclopedia of Genetics (2nd ed.), San Diego: Academic Press, pp. 358–361, ISBN 978-0-08-096156-9, retrieved 2022-04-06
- ^ Liscum, Emmanuel; Askinosie, Scott K.; Leuchtman, Daniel L.; Morrow, Johanna; Willenburg, Kyle T.; Coats, Diana Roberts (January 2014). "Phototropism: Growing towards an Understanding of Plant Movement[OPEN]". The Plant Cell. 26 (1): 38–55. doi:10.1105/tpc.113.119727. ISSN 1040-4651. PMC 3963583. PMID 24481074.
- ^ Chen, Rujin; Rosen, Elizabeth; Masson, Patrick H. (1 June 1999). "Gravitropism in Higher Plants". Plant Physiology. 120 (2): 343–350. doi:10.1104/pp.120.2.343. PMC 1539215. PMID 11541950.
- ^ Sato, Ethel Mendocilla; Hijazi, Hussein; Bennett, Malcolm J.; Vissenberg, Kris; Swarup, Ranjan (2015-04-01). "New insights into root gravitropic signalling". Journal of Experimental Botany. 66 (8): 2155–2165. doi:10.1093/jxb/eru515. ISSN 0022-0957. PMC 4986716. PMID 25547917.
- ^ a b Kim, Keunhwa; Shin, Jieun; Lee, Sang-Hee; Kweon, Hee-Seok; Maloof, Julin N.; Choi, Giltsu (2011-01-25). "Phytochromes inhibit hypocotyl negative gravitropism by regulating the development of endodermal amyloplasts through phytochrome-interacting factors". Proceedings of the National Academy of Sciences. 108 (4): 1729–1734. Bibcode:2011PNAS..108.1729K. doi:10.1073/pnas.1011066108. ISSN 0027-8424. PMC 3029704. PMID 21220341.
- ^ a b Meškauskas, A., Novak Frazer, L. N., & Moore, D. (1999). "Mathematical modelling of morphogenesis in fungi: a key role for curvature compensation ('autotropism') in the local curvature distribution model". New Phytologist. 143 (2): 387–399. doi:10.1046/j.1469-8137.1999.00458.x. PMID 11542911.
{{cite journal}}
: CS1 maint: multiple names: authors list (link) - ^ Meškauskas A., Jurkoniene S., Moore D. (1999). "Spatial organization of the gravitropic response in plants: applicability of the revised local curvature distribution model to Triticum aestivum coleoptiles". New Phytologist. 143 (2): 401–407. doi:10.1046/j.1469-8137.1999.00459.x. PMID 11542912.
{{cite journal}}
: CS1 maint: multiple names: authors list (link) - ^ Wolverton, Chris; Paya, Alex M.; Toska, Jonida (2011-04-01). "Root cap angle and gravitropic response rate are uncoupled in the Arabidopsis pgm-1 mutant". Physiologia Plantarum. 141 (4): 373–382. doi:10.1111/j.1399-3054.2010.01439.x. ISSN 1399-3054. PMID 21143486.