User:Slicky/Microscopy in science
A microscope (Greek: micron = small and scopos = aim) is an instrument for viewing objects that are too small to be seen by the naked or unaided eye. The science of investigating small objects using such an instrument is called microscopy, and the term microscopic means minute or very small, not easily visible th the unaided eye. In other words, requiring a microscope to examine.


The most common type of microscope—and the first to be invented—is the optical microscope. This is an optical instrument containing one or more lenses that produce an enlarged image of an object placed in the focal plane of the lens(es).
Simple optical microscope
It is the first microscope to be invented in 1590s. After that there is varied changes occurs in the design in this simple microscope. Routinely it is most commonly used.
Compound optical microscope
The diagram below shows a compound microscope. In its simplest form—as used by Robert Hooke, for example—the compound microscope would have a single glass lens of short focal length for the objective, and another single glass lens for the eyepiece or ocular. Modern microscopes of this kind are usually more complex, with multiple lens components in both objective and eyepiece assemblies. These multi-component lenses are designed to reduce aberrations, particularly chromatic aberration and spherical aberration. In modern microscopes the mirror is replaced by a lamp unit providing stable, controllable illumination.

1. ocular lens or eye-piece
2. objective turret, or nosepiece
3. objective lenses
4. coarse adjustment knob
5. fine adjustment knob
6. object holder or stage
7. mirror
8. diaphragm and condenser

History of the microscope
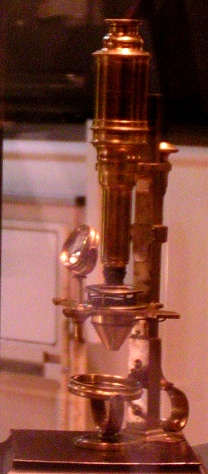
It is impossible to say who invented the compound microscope. Dutch spectacle-makers Hans Janssen and his son Zacharias Janssen are often said to have invented the first compound microscope in 1590, but this was a declaration by Zacharias Janssen himself halfway through the 17th century. The date is certainly not likely, as it has been shown that Zacharias Janssen actually was born around 1590. Another favorite for the title of 'inventor of the microscope' was Galileo Galilei. He developed an occhiolino or compound microscope with a convex and a concave lens in 1609. Galilei´s microscope was celebrated in the ´Lynx academy´ founded by Federico Cesi in 1603. Francesco Stelluti´s drawing of three bees were part of pope Urban VIII´s seal, and count as the first microscopic figure published (see Stephen Jay Gould, The Lying stones of Marrakech, 2000). Christiaan Huygens, another Dutchman, developed a simple 2-lens ocular system in the late 1600's that was achromatically corrected and therefore a huge step forward in microscope development. The Huygens ocular is still being produced to this day, but suffers from a small field size, and the eye relief is uncomfortably close compared to modern widefield oculars.
Anton van Leeuwenhoek (1632-1723) is generally credited with bringing the microscope to the attention of biologists, even though simple magnifying lenses were already being produced in the 1500's, and the magnifying principle of water-filled glass bowls had been described by the Romans (Seneca). Van Leeuwenhoek's home-made microscopes were actually very small simple instruments with a single very strong lens. They were awkard in use but enabled van Leeuwenhoek to see highly detailed images, mainly because a single lens does not suffer the lens faults that are doubled or even multiplied when using several lenses in combination as in a compound microscope. It actually took about 150 years of optical development before the compound microscope was able to provide the same quality image as van Leeuwenhoek's simple microscopes. So although he was certainly a great microscopist, van Leeuwenhoek is, contrary to widespread claims, certainly not the inventor of the microscope.
The parts of the microscope
At the top of the microscope is the eyepiece or ocular - a cylinder containing two or more lenses to bring the image to focus for the eye. The eyepiece is inserted into the top end of the body tube. This design allows for different eyepieces to be inserted to give different magnifications. Typical values for eyepieces include X5, X10 and X20. At the lower end of the microscope tube are one or more objective lenses mounted on a circular nose piece. Each objective lens is cylindrical and is screwed into to the nose piece. Typical values of objectives are x5, x10,x20, x40, x80 and x100. Below the objectives is the stage which supports the specimen being viewed. In the centre of the stage is a circular hole through which light shines to illuminate the specimen. Below the stage, the light is provided and controlled in a variety of ways. At its simplest, daylight is directed via a mirror. Most microscopes, however, have their own controllable light source that is focused through an optical device called a condenser with diaphragms and filters available to manage the quality and intensity of the light.
The whole of the optical assembly is attached to a rigid arm which in turn is attached to a robust U shaped foot to provide the necessary rigidity. The arm is usually able to pivot on its joint with the foot to allow the viewing angle to be adjusted. Mounted on the arm controls for focusing are usually placed, typically a large knurled wheel to control coarse focusing together with a smaller knurled wheel to control fine focusing.
Compound optical microscopes can produce a magnified image of a specimen up to 1000× and, at high magnifications, are used to study thin specimens as they have a very limited depth of field. Typically they are used to examine a smear, a squash preparation, or a thinly sectioned slice of some material. With a few exceptions, they utilize light passing through the sample from below and special techniques are usually necessary to increase the contrast in the image to useful levels (see contrast methods). Typically, on a standard compound optical microscope, there are three objective lenses: a scanning lens (5×), low power lens (10×), and high power lens (40×). Advanced microscopes often have a fourth objective lens, called an oil immersion lens. To use this lens, a drop of oil is placed on top of the cover slip, and the lens moved into place where it is immersed in the oil. An oil immersion lens usually has a power of 100×. The actual power or magnification is the product of the powers of the ocular (eyepiece), usually about 10×, and the objective lens being used.
To study the thin structure of metals (see metallography) and minerals, another type of microscope is used, where the light is reflected from the examined surface. The light is fed through the same objective using a semi-transparent mirror.
How a microscope works
The optical components of a modern microscope are very complex and for a microscope to work well, the whole optical path has to be very accurately set up and controlled. Despite this , the basic optical principles of a microscope are quite simple.
The objective lens is, at its simplest, a very high powered magnifying glass i.e. a lens with a very short focal length. This is brought very close to the specimen being examined so that the light from the specimen comes to a focus about 160 mm inside the microscope tube. This creates a virtual and enlarged image of the subject. This image is inverted and can be seen by removing the eyepiece and placing a piece of tracing paper over the end of the tube. By careful focusing a rather dim image of the specimen, much enlarged can be seen. It is this virtual image that is viewed by the eyepiece lens that provides further enlargement.
In most microscopes, the eyepiece is a compound lens, which is made of two lenses one near the front and one near the back of the eyepiece tube forming an air separated couplet. In many designs, the virtual image comes to a focus between the two lenses of the eyepiece, the first lens bringing the virtual image to a focus and the second lens enabling the eye to focus on the image.
In all microscopes the image is viewed with the eyes focused at infinity. Headaches and tired eyes after using a microscope are usually signs that the eye is being forced to focus at a close distance rather than at infinity.
Stereomicroscope

The stereo or dissecting microscope is designed differently from the diagrams above, and serves a different purpose. It uses two separate optical paths with two objectives and two eypieces to provide slightly different viewing angles to the left and right eyes. In this way it produces a three-dimensional (3-D) visualisation of the sample being examined.
The stereo microscope is often used to study the surfaces of solid specimens or to carry out close work such as sorting, dissection, microsurgery, watch-making, small circuit board manufacture or inspection, and the like.
Great working distance and depth of field here are important qualities for this type of microscope. Both qualities are inversely correlated with resolution: the higher the resolution (i.e., magnification), the smaller the depth of field and working distance. A stereo microscope has a useful magnification up to 100×. The resolution is maximally in the order of an average 10× objective in a compound microscope, and often much lower.
The stereo-microscope should not be confused with ordinary compound microscopes equipped with a binocular eyepieces. In these microscopes both eyes can see the image but the binocular head provides greater viewing comfort and slightly better appearance of resolution. However the image in such microscopes remains monocular.
Special designs
Other types of optical microscope include:
- the inverted microscope for studying samples from below; useful for cell cultures in liquid;
- the student microscope designed for low cost, durability, and ease of use; and
- the research microscope which is an expensive tool with many enhancements.
- the petrographic microscope whose design usually includes a polarizing filter, rotating stage and gypsum plate to facilitate the study of minerals or other crystalline materials whose optical properties can vary with orientation.
Optical resolution
A lens magnifies by bending light (see refraction). Optical microscopes are restricted in their ability to resolve features by a phenomenon called diffraction which, based on the numerical aperture (NA or ) of the optical system and the wavelengths of light used (), sets a definite limit (d) to the optical resolution. Assuming that optical aberrations are negligible, the resolution (d) is given by:
Usually, a of 550 nm is assumed, corresponding to green light. With air as medium, the highest practical is 0.95, and with oil, up to 1.5.
Due to diffraction, even the best classic optical microscope is limited to a resolution of 0.2 micrometres.
Naming guideline for microscopes
The naming of microscopes follows in an increasing order to the importance of an underlying fundamental microscopy technique, with the source/illumination principle usually being the most important for the classification of a microscope (e.g. light,fluorescence,x-ray...). For example in the Confocal Laser Scanning Microscope the source/illumination technique is a positionable laser-scanning device, which primarily classifies the microscope.
There is no standardization nor convention existant, however the naming of microscopes by their underlying techniques usually follow those guidelines. Thus for instance the Confocal Laser Scanning Microscope/CLSM (~75% google-hits) is also known as the Laser Scanning Confocal Microscope/LSCM by a web-wide percentage of 25% (according to google).
Types and classification of microscopes

Foremost due to importance in todays technology to observe micro-pico scale structure and processes a vastness of microscope designs and implementations, improvement exists all of which are usually employed as soon as the first functional prototype is available. Thus using acronyms as abbreviations makes only sense in a context where it is clear which microscope is referred to otherwise, especially the three letter acronyms are ambiguous.
The classification of a microscope is based upon its underlying fundamental field of interest e.g. magnetic field, electric field or electromagnetic field. Intrinsically it is not yet possible to probe by other fundamental forces such as gravitation or the strong/weak nuclear force. In fact the fundamental forces except electromagnetism are heavily disputed, and some unified theories state the existence of only one fundamental force: electromagnetism[1]. It may be possible in the future to probe for subatomic particles, as our understanding of fundamental physical principles progresses. So far the uncertainty principle (HUP) can seemingly be violated in the frequency/energy-time uncertainty relation when there is a priori knowledge about the class of parameterized functions used [11]. However the uncertainty principle cannot be overcome in an "underparameterized" system, which in this case holds true so far for any quantum system, thus limiting further progress "until we can define the nature of the atom enough to complete the grand unification theory(GUT) and fully explain quantum mechanics in mathematical terms"[10]. Moreover it takes time to realize the potential of certain technological advancements like bose einstein condensates(BEC) (e.g. the full potential of the LASER was in it's early days unknown, and represents now in the 21st century one of the most important technologies spanning over all natural sciences)[2]
Magnetic field based
Technology
- Sensor: Low temperature superconducting quantum interference
device (SQUID); state of the art as of 2000 are 6nm Semiconductor SQUIDs; story
- Spatial resolution: 1 µm for single dipole sources
- Commercially available:Yes (Tristan Technologies, IBM,...)
Introduction
A Scanning Magnetic Flux Microscope is a device that can map magnetic fields with a spatial resolution in the micrometre range. The detector uses a superconducting quantum interference device to sense tiny magnetic fields from a sample which moves back and forth beneath the SQUID in steps according to the resolution of the device. The scientific uses of the magnetic force microscope include prospecting for the characteristic fields emanating from microscopic nuggets of superconductor buried inside otherwise non-superconducting samples. The microscope can also be used to image poorly-magnetic materials such as thin copper patterns on printed circuit boards by measuring the faint magnetic fields that arise from eddy currents induced in the copper or other materials.(R.C. Black et al., 3 January 1994, Applied Physics Letters.)
Application
- micro-current distributions
- vortex motion in superconductors
- traces on a circuit board ormulti-chip module
- weak electric currents in semiconductors
- integrated circuits
- magnetic domains
- transient magnetic properties
- magnetic susceptibility
- magnetic hysteresis
Types/Names
- Magnetic force microscope/Magnetic field microscope (MFM)
- Ultra High Resolution Scanning SQUID Microscope (UHRSSM)
- Scanning Magnetic Flux Microscope
- Scanning SQUID microscope
- Nuclear Magnetic Resonance Microscope (NMRM) / MRM
- Magnetic resonance force microscope (MRFM)
- Combined Optical Magnetic Resonance Microscope
Electric field based
- Atom probe based microscopy/tomography(AP/APT) are not limited by diffraction, but only by the size of the probe-sample interaction volume (i.e., point spread function). They all are Scanning probe microscopes, and thus rather slow.
- Scanning tunneling microscope
- Atomic force microscope
- Electronic Force Microscopy
- Lateral Force Microscopy (LFM)
- Ultrasonic Force Microscopy (UFM)
- Atomic Force Acoustic Microscopy (AFAM)
- Exchange Force Microscopy EFM
- Friction Force Microscopy (FFM)
- Ultra high resolution Field Emission Gun Scanning Electron Microscope (FEG SEM)
- High Pressure Scanning Tunnelling Microscopy (HP-STM)
- Biochemical AFM BAFM
- Exchange Force Microscopy
- Friction Force Microscopy (FFM)
- High Pressure Scanning Tunnelling Microscopy (HP-STM)
- Piezo-Force Microscopy (PFM)
- Heterodyne Ultrasonic and Electrostatic Force Microscopy (HUEFM)
- Scanning Surface Potential Microscopy (SSPM)
- Scanning Spreading Resistance Microscope (SSRM)
- Rapid Atomic Force Microscope
- Kelvin probe force microscope (KPFM) /surface potential microscopy
- PoSAP microscopy (position sensitive atom probe
- 1DAP (one dimensional atom probe)
- 3DAP (three dimensional atom probe)
- LEAP (Local-Electrode Atom-Probe) microscopy
- SVM scanning voltage microscope
- Scanning capacitance microscope SCM
- Electrostatic force microscope EFM
- Force modulation microscope FMM
- Scanning gate microscope SGM
- Field ion microscope FIM
- Field emission microscope FEM
- Field Emission Energy Filter Electron Microscope EF-FEM
- Electrochemical scanning tunneling microscope
- LEEM Low Energy Electron Microscopy
- Spin-Polarized energy filtered LEEM (SPE-LEEM): for chemical analysis
- SPLEEM (Spin-Polarized Low Energy Electron Microscopy): for the study of magnetic materials
- LEED Low Energy Electron Diffraction Microscopy
- SPLEED Spin-Polarized Low Energy Diffraction Microscopy
Articles/Information
- There is a concise article by Dr. Huey differentiation the use and application of various Atomic force microscopes available here.
Electromagnetic field based
For the classification according to the wavelength, see electromagnetic spectrum.
Further classification could be made by pulsation and duration of Laser light, wavelength/energy, linearity/non-linearity, and foremost whether or not vital microscopy can be carried out.
Atom beam
- Atom beam microscope: generic term in analogy to electron (beam) microscope. Next generation nanoscopes featuring subnanometer wavelengths and with intert/uncharged beam entirely non-destructive and non-invasive in-situ studies.
- Scanning Helium Microscope (SHeM)
- Scanning Helium Ion Microscope: next-gen microscope, high-res microscopy,low-energy sec. e-beam, high source brightness,shorter wavelength
- Focused ion beam microscopy(FIB)
- Dual-column ion/electron beam systems, Dual column SEM/FIB: combines ultra-high resolution field emission scanning electron microscopy (SEM) and focused ion beam (FIB) etch and deposition for nanoscale prototyping, machining, 2-D and 3-D characterization, and analysis.
- See also:
- Helium atom scattering (HAS-detail)
- Low energy electron diffraction (LEED-detail)
- [1] Nanoscale prototyping at 1nm, with importance of polymer cross-links (~1 nanometer) and chemical bonds (~0.2 nanometers): "Molecular scale resolution achieved in polymer nanoimprinting technique"
Electron beam
- Electron microscope
- Cryo-electron microscopy
- Energy filtered transmission electron microscopy(EF-TEM): Image is formed using only electrons that have suffered an energy loss characteristic of the element of interest
- High Resolution Transmission Electron Microscopy(HR-TEM): Provide structural information at better than 0.2 nm spatial resolution
- PEEM (photoemission electron microscope)
- SEM Scanning electron microscope
- SCEM Scanning Confocal Electron Microscope
- TEACM Transmission Electron Aberration-corrected Microscope or ACTEM
- TEM Transmission electron microscopy: when used in contrast to STEM, TEM often means Tunneling Electron Microscope
- STEM Scanning transmission electron microscopy: can also refer to Scanning Transmission Electron Module
- Scanning transmission electron microscope with an X-ray energy dispersive spectrometer (STEM-XEDS)
- (Quantitative) Environmental Cell - Transmission Electron Microscopy QEC-TEM / EC-TEM
- 3DEM 3D-Electron microscopy, electron tomography
- LVSEM: Low Voltage Scanning Electron Microscopy
- TEAM Transmission Electron Aberration-corrected Microscope (article1)
- Intermediate Voltage Electron Microscope IVEM /IVSEM
- ESEM: Environmental Scanning Electron Microscopy: a form of electron microscopy that can be carried out under atmospheres rather than vacuum
- STXM / SXTM Scanning Transmission X-ray Microscopy: synchrotron-based soft X-ray transmission Microscope
- Cryo scanning transmission X-ray microscope
- Laser plasma-based soft x-ray microscopy LPXM: x-ray imaging with the quality of synchrotron-based microscopes
- see also:
Deep ultraviolet (230 nm to 350nm)
- Deep Ultraviolet microscope: Research stage; DUV light does not transmit through ordinary glass, and hence cannot be observed with standard microscope optics.
- Ultraviolet microscopy: Research stage; Microscopes with a UV source for excitation of fluorophores are usually not referred to as UV microscopes
Infrared
- Infrared microscope
- Infrared Near-Field Microscopy / IR-NSOM
- Thermal emission microscopy / Infrared emission microscopy: measures the spatial distribution of temperature in a sample; non-contact optical microscopy technique collecting mid-infrared photons emitted by the sample
Visible light (380 to 780 nm)
Continuous light
- Brightfield microscope
- Darkfield microscope
- Polarized Light Microscopy
- Stereomicroscope
- Ultramicroscope
- Inverted microscope
- Cathodoluminescence microscope
- DIC microscope (differential interference contrast)
- Hoffman Modulation Contrast Microscope
- Phase contrast microscope, see Frits Zernike
- Adaptive Scanning Optical Microscope (ASOM): Sucessor of SOMS *Scanning Optical Mosaic Scope (SOMS): large-field-of-view optical microscope using scanning mirrors
- Spinning disk confocal microscope (SDCM): article links to brochures
Fluorescence
- Fluorescence microscope: Classic fluorescence microscope with continuous UV emitter
- Fluorescence speckle microscopy
Phosphorescence
- Phosphorescence Microscope: Various techniques exist to acquire phosphorescence data for specific studies
Discrete/Coherent light
- Two-photon excitation microscopy
- Photonic force microscope
- Two-Beam Interferometry microscope
- Multi-Beam Interferometry microscope
- Reflection confocal microscopy
- Backscatter-enhanced reflection confocal microscopy
- Confocal laser scanning microscopy / LSCM (Laser Scanning Confocal Microscopy)
- 4Pi microscopy
- 4Pi STED microscopy
- CARS microscopy
- NSOM / SNOM Near field scanning optical microscopy
- TENOM Tip enhanced nonlinear optical microscopy
- Multifocal Multiphoton Microscopy (MMM)
- STED microscopy
- I²M: interference illumination microscopy
- I³M: incoherent interference illumination microscopy
- I5M: The two techniques,I²M to further sharpen the pattern and I³M can be used together as I5M
- 4Pi-I5M: The result with both 4Pi and I5M is 7-fold improvement in resolution in the z axis, providing better axial than lateral resolution (Hell and Stelzer, 1992; Gustafsson et al., 1999; Egner et al., 2002).
- SSIM (Saturated structured illumination microscopy)
- PLAP Pulsed Laser Atom Probe microscope
Fluorescence
- Epiflourescence microscopy
- Total internal reflection fluorescence microscope
- Fluorescence recovering after photobleaching (FRAP) microscope
- Fluorescence resonance energy transfer (FRET) microscope
Radio frequency
- Scanning Near-Field RF Microscopy (RF-NSOM)
- Scanning radar microscope: a radar configuration for microscale meteorological research
Microwave
- Scanning Near-Field Microwave Microscopy (MW-NSOM/SNMM)
Notice: Although those microscopes rely upon Masers, the term (e.g. maser microscope) is not used. Therefore use 'coherent microwave' instead.
X-Ray
- X-ray microscope
- Soft x-ray microscopy
- [X-Ray Absorption Fine Structure] (XAFS) Microscope
- Extended X-ray Absorption Fine Structure (EXAFS)
- Near Edge X-ray Absorption Fine Structure (NEXAFS) microscopy:used to distinguish a variety of polymer species
- Quick Extended X-ray Absorption Fine Structure (QEXAFS)
- x-ray free electron laser (XFEL) microscopy (see also FEL)
- 3D x-ray microscopy / High resolution 3-D x-ray microscopy
- 2D x-ray fluorescence microscopy
- Vacuum Ultraviolet Free-Electron Laser (VUV-FEL) at DESY
See also
- ANKA is the synchrotron light source of the Forschungszentrum Karlsruhe, providing light from hard X-rays to the far-infrared for research and technology.
Gamma ray (wavelength >=1pm)
- Gamma ray microscope: So far a hypothetical microscope invented by Heisenberg
Other / microanalysis
- Ultrasound backscatter microscope (UBM)
- http://www.asu.edu/clas/csss/chrem/techniques/EDX.html Energy Dispersive X-ray Microanalysis (EDX)
- Spectroscopic / Diffraction Microanalysis:
- Electron Energy Loss Spectroscopy (EELS)
- Selected Area Electron Diffraction (SAED): used amongst others in TEMs for phase identification
- STEM X-ray Microanalysis: See STEM
- CBED: Convergent-beam electron diffraction: essential method for the analysis of crystalline materials at the nanometer level
- Nanodiffraction:special form of CBED
- EELS: Electron Energy Loss Spectroscopy
- PEELS: [http://www.asu.edu/clas/csss/chrem/techniques/EELS.html Parallel electron energy-loss spectrometer
]
Generic
- Digital microscope e.g. MIC-D
- Nuclear microscopy
- Virtual microscope: Any educational/training related project with the objective to emulate a certain microscope
- TPM: TelePresence Microscopy: allows remote viewing and operation using Network protocols
- Lorentz microscopy
Common Classifications
- HREM: High Resolution Electron Microscopy
- SPM: Scanning Probe Microscope
- EF:Energy Filtered Imaging
- ECM: Environmental Cell Microscopy
Common acronyms
Acronym | Meaning |
---|---|
AAS | Atomic Absorption Spectroscopy |
ACF | absorption correction factor |
ADC | analog to digital converter |
AED | Atomic Emission Detector |
AEM | analytical electron microscope/microscopy |
AES | Atomic Emission Spectroscopy |
... | |
... | |
XPEEM | X-ray Photoemission Electron Microscopy |
XPLEEM | X-ray Photoemission and Low Energy Electron Microscopy |
XPS | X-ray photoelectron spectroscopy |
XRD | X-ray diffraction |
XRF | X-ray Fluorescence |
ZAF | atomic number, absorption, fluorescence correction |
See also
- Angular resolution
- Microscope image processing
- Microscope slide
- Microscopy
- Microscopy laboratory in: A Study Guide to the Science of Botany at Wikibooks
- Telescope
- Condensed Matter Physics
- Diffusion
- Low Temperature
- Luminsecence
- Molecular Orientation
- Nanoscale Plasma
- Nanoscience
- Nanotechnology
- Nanotribology
- Single molecule fluorescence
- Spectroscopy
- Surface science
References
- Max Planck Research Group
- Nanotube helium sensors could bring atom beam microscope
- [F1]:"Phase-coherent amplification of atomic matter waves" (Nature 1999/12, S. INOUYE, T. PFAU, S. GUPTA, A. P. CHIKKATUR, A. GÖRLITZ, D. E. PRITCHARD & W. KETTERLE )
- D.A. MacLaren, H. T. Goldrein, B. Holst and W. Allison, Phase-stepping optical profilometry of atom mirrors, J. Phys. D., 36, 1842-1849, 2003
- D. A. MacLaren, B. Holst, D. Riley and W. Allison, Focusing elements and design considerations for a scanning helium microscope (SHeM), SurfaceReview and Letters, 10, 249-255, 2003
- Why use a Stem and not a Tem?
Notes
- ^ New Scientist; et al. (12/10/2005). "Editorial". Retrieved 2006-04-19.
{{cite web}}
: Check date values in:|date=
(help); Explicit use of et al. in:|author=
(help) - ^ Colorado University. "Science 2000 Projcet". Retrieved 2006-04-19.
External links
- Directory of Microscopy & Microanalysis Meetings/ShortCourses/Conferences 2006
- A virtual polarization microscope (requires Java)
- How To Buy A Microscope
- Micscape - a monthly magazine directed towards the amateur microscopist
- Microscopy
- the optics of the microscope
- Optical microscopy primer
- Royal Microscopical Society
- The Microscope - quarterly journal
- Antique German microscopes history of continental microscopes illustrated with 2000 photos (in German)
- Early American made microscopes Antique American made microscopes and the makers.
- Some Early Microscopes from the Optical Institute in Wetzlar Microscope history.
- Mikroskop-Museum The history of the microscope from the beginning to an high-end research instrument in material- and lifescience (in German).